Robert Blick's research group
Microwave Spectroscopy on a Double Quantum Dot using a Josephson oscillator as an on-chip source
We present a timely technique to perform microwave spectroscopy
on lateral quantum dots. The quantum dots are defined in a two dimensional electron
gas (2DEG) of a GaAs/AlGaAs heterostructure with a mobility of
m = 8*105 cm2/Vs and a density
of nS = 1.7*1011 cm-2 at a base temperature of Tbath
~ 35 mK. On the surface of this heterostructure gate electrodes of Au are
evapourated. By applying negative voltages to the gates the 2DEG underneath is depleted
and the geometry of the gate structure is transfered to the 2DEG. Thus, we can form
a serial double quantum dot which is isolated to the 2DEG via tunnel barriers (see
inset of Fig. 1.).
For performing microwave spectroscopy an electromagnetic radiation in the frequency range of f = 0.5-100 GHz is coupled into the dot system and the variation of the current through the quantum dot is detected. Generally, electron transport through a quantum dot is characterised by the Coulomb blockade [1]. Adding extra electrons to a quantum dot costs a charging energy which can be higher than the thermal energy of the electrons at the Fermi-level in the 2DEG. Only at discrete levels, when the charging energy coincides with the energy which is provided by the electrons in the 2DEG, current is possible, otherwise the current is blockaded. In the presence of an external time-varying field, the Tien-Gordon theory [2] predicts electron transport through the quantum dot meanwhile photons are absorped respectively emitted. This effect is called photon assisted tunneling [3].
So far, only external microwave sources have been used [4,5]. In which case the microwaves are coupled via waveguides respectively coaxial lines and an antenna into the quantum dots. Here, we present an on-chip approach with a long tunnelconact as a microwave source. Generating the microwaves in close vicinity to the dots provides three advantages. Firstly, all lines to the system under test are dc-lines and thus, can heavily been filtered. Secondly, the probed system is located within the near-field of the radiation source. In our set-up the distance between the source and the quantum dot is about 3 mm, the wavelength for a radiation of 10 GHz is l ~ 3 cm, see Fig. 1. Last, but not least we need only an ordinary 9 V battery to perform this sophisticated experiment! As a microwave generator we used a Nb/Al-AlOx /Nb tunnelcontact. The silicon chip bearing the Josephson junction is glued on top of the heterostructure with the double dot by photo resist (see Fig. 1.).
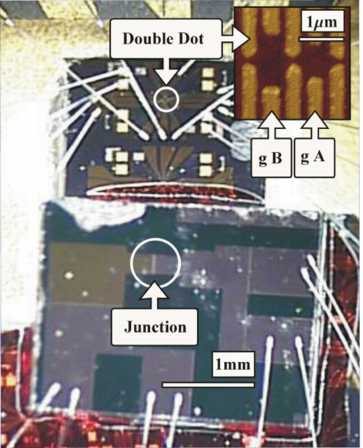
Figure 1. See text for more details
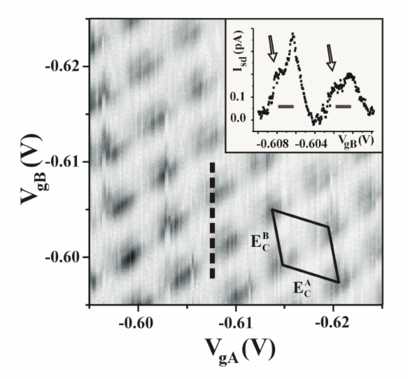
Figure 2. See text for more details
In the inset of Fig. 2. the current through the double dot is plotted over the voltage VgB which is applied to gate B (see inset of figure 1.), e.g. at a value of VgB ~ -606.5 mV electron transport through the structure is possible, hence there is a current of about I ~ 0.35 pA. Furthermore, the microwave induces a sideband at VgB ~ -608 mV. The difference in voltage corresponds to a frequency of f = 10 GHz. Sweeping in addition the voltage VgA, which is applied to gate A (see inset of Fig.1.) results in the charging diagram which is depicted in Fig.2. Black area refers to a current of I > 0.5 pA and white area to I < 0 pA. The broken line corresponds to the linegraph in the inset. Clearly, one can see the induced sidepeaks over the whole range of the gate voltages [5].
[1] L.P. Kouwenhoven et al., Z. Phys. B 85, 367 (1991).
[2] P.K. Tien and J.P. Gordon, Phys. Rev. 129, 647 (1963).
[3] L.P. Kouwenhoven et al., Phys. Rev. Lett. 73, 3443 (1994); R.H. Blick
et al., Appl. Phys. Lett. 67, 3924 (1995).
[4] R.H. Blick et al., Phys. Rev. Lett. 81, 689 (1998); T.H. Oosterkamp
et al., Nature 395, 873 (1998).
[5] A.W. Holleitner et al., New Journal of Physics 2, 2.1-2.7 (2000).
Preparation and characterization of suspended nanostructures in Silicon-On-Insulator
We want to investigate the mechanical properties of free standing nanowires as well as of more complex structures in the linear and in the nonlinear regime. The devices are fabricated using high-resolution low-energy electron beam lithography, evaporation of metallic films and dry and wet etching techniques. We therefore realized suspended Au/NiCr/Si hybrid systems measuring down to 100 nm (width) x 50 nm Si + 50 nm Au (thickness) x 500 nm (length). The expected resonance frequency of such devices is around 500 MHz. The measurement setup consists of a cryostat, equipped with a superconducting coil allowing to generate magnetic fields up to 12 Tesla. The sample is mounted in a suitable holder and put in the magnetic field while an alternate current is fed in the sample itself. The ac current (of variable frequency) flowing in the suspended nanowire in the presence of the magnetic field is responsible for generating a Lorentz force acting on the wire. When this excitation matches the mechanical eigenfrequency of the wire, resonant motion is detected by the network analyzer as a change of the reflected power.
Apart from studying nanomechanics, our aim is to study the interaction of single electrons with phonon modes, which should be possible thanks to the thermal insulation between the suspended nanowire and the substrate. We have observed features in the nonlinear conductance of a suspended silicon nanowire (not a hybrid system as before, but a wire fabricated out of highly doped silicon), which can be explained in terms of discrete phonon modes when the phonon dominant wavelength becomes comparable to the wire dimensions.
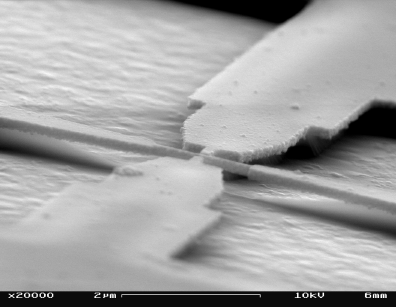
Complex Photoconductance Measurements on a Single Quantum Dot
Spectroscopy on quantum dots is commonly performed either by non-linear transport or by microwave measurements. Ordinary linear transport, i.e. under a small forward bias Vds between source and drain contact and without microwave irradiation, only involves quantum dot ground states. In the non-linear case, by applying a finite bias across the "artificial atom", also excited quantum dot states can participate in transport. Furthermore, in the presence of a microwave field electrons can absorb or emit photons and thus reach excited quantum dot states otherwise not available in linear transport -- a phenomenon known as photon-assisted tunneling (PAT).
In a combination of the two methods described above, we conduct photoconductance measurements on a small quantum dot both in the linear and non-linear transport regime. The principle of this method is that by using two phase-locked microwave sources with a slight frequency offset and detecting the photoconductance signal at the offset frequency, the amplitude of the photoconductance and the relative phase between the photocurrent and the microwave beat can be obtained. There are two alternatives of this method as shown in Fig.1.
- When two coherent continuous microwave sources are mixed directly and fed to the antenna, we get a continuous microwave radiation. We are able to study resonant tunneling and PAT through the ground state as well as through excited states. PAT in the non-linear regime can also be resolved. We are thus not limited by the broadening of the conductance resonances due to finite bias.
- If the non-linear transmission lines (NLTLs) are applied to multiple the base frequency (f) and hence generate wideband millimeter-wave radiation, the radiation is emitted in form of pulses frequencies of n*f. Since we detect these harmonics with a lock-in amplifier, we can perform broadband spectroscopy on the dot system without changing the heat load in contrast to conventional tuned sources, such as Gunn diodes. Most importantly, due to the pulsed radiation of we observe time dependent variations of the quantum dot's capacitance, which is strongly related to competition between the pumping rate (f) and the escape rate of the electron tunnel to/off the artificial atom. Combined with the photoconductance amplitude, the relative phase seems to reveal the nature of different types of transport through the atom.