Research report 2007
- Introduction
- Electronic properties
- Optical and optoelectronical phenomena in nanosystems
- Micropatterned Electrostatic Traps for Indirect Excitons in Coupled GaAs Quantum Wells
- Optically induced charge transport through submicron channels
- Statistics of quantum dot exciton fine structure splittings and their polarization dependence
- Rabi splitting and ac-Stark shift of a charged exciton
- Optical detection of single electron spin resonance in a quantum dot
- The Nonlinear Fano Effect
- Dimensionally constrained D’yakonov Perel’ spin relaxation in n-InGaAs channels: transition from 2D to 1D
- A photosynthetic reaction center covalently bound to carbon nanotubes for nanoscale optoelectronic circuits
- Nanomechanical systems
- Nano-mechanical electron transport at 20 Kelvin
- An optomechanical system at the nanoscale
- Bose-Einstein condensates coupled to micro- and nanomechanical resonators on an atom chip
- Nanomechanical resonators under high tensile stress
- Statistical analysis of arrays of pillar resonators
- A suspended single-walled carbon nanotube resonator
- Nanobioscience
- Diploma theses in 2007
- PhD theses in 2007
- Guest scientists in 2007
- Group members in 2007
- Publications in 2007
- Invited talks in 2007
- Senior scientists of cooperating groups
- Financial support in 2007
Introduction
Nanoscale devices and systems continue to be the major subject of our research. In our work we combine the realization and study of top-down fabricated devices employing techniques derived from semiconductor processing with the exploration of bottom-up assembled systems made via chemical and biochemical routes. Our goals range from a deeper fundamental understanding of physical behavior in the nanometer regime to the realization of novel devices, functions, and systems utilizing a variety of nanotechnologies.
Our studies of electronic properties of nanoscale systems aim at exploring the effects of quantum confinement and many-body interactions on electronic behavior and their potential for applications in processing information. One ongoing major research theme are ways to implement and control quantum bits on the basis of solid state electronic and optical devices and thus lay the ground work for a future quantum information technology. In part this research is embedded in the SFB 631 - a long-term research platform on " Solid-State Based Quantum Information Processing", supported by the German Science Foundation (DFG) since July 2003. In addition we participate in a research program on "Nanoelectronic semiconductor structures for the quantum information technology" (NanoQuit) funded since January 2005 by the German Ministry for Education and Research (BMBF) with research on "Quantum control in suspended semiconductor nanostructures" (QuBridge). These experiments are complemented by studies of electronic transport in molecular devices including carbon nanotubes and organic compounds.
Optical characterization and control of individual self-assembled quantum dots continue to be an important part of our activities in the field of nanooptics. This work is based on a continuous collaboration with the groups of Pierre Petroff at UC Santa Barbara and Richard Warburton at Heriot-Watt University in Edinburgh and aims at studying and controlling spin and charge quantum states with optical techniques. The dynamics of long-living excitons created in double quantum wells and moving in a controlled artificial potential landscape is another aspect of our nanooptical studies. These experiments aim at understanding many-body interactions in excitonic condensates and at the realization of Bose-Einstein condensation in excitonic systems.
In the nanometer regime electronic, mechanical and optical properties become often inseparably connected. This motivates our experiments on Nano-Electro-Mechanical Systems, in short NEMS. Ways to efficiently drive such nanoscale resonators by electrically, magnetically, mechanically as well as optically induced forces are one current research aspect. Sensing the mechanical motion with nanometer resolution via capacitance, current or optical reflection is another basic theme of ongoing research. Those basic studies of mechanical nanosystems are complemented by the investigation of their coupling to single electron transistors, high finesse optical cavities, as well as Bose-Einstein condensates.
The investigations in the area of nanobioscience, directed by Friedrich Simmel, who headed a DFG-funded Emmy-Noether junior group, successfully aim at creating artificial nanomachines by combining bottom-up biochemical assembly routes with top-down fabrication. DNA-templated and genetically controlled assembly is combined with top-down fabrication to realize and study chemically and electrodynamically driven nanodevices and -machines. The recognition received by his outstanding research is not only reflected by many invited talks at international meetings but also by several offers of tenured professor positions Friedrich received, finally accepting a Full Professor position at the Technical University of Munich, where he started in November 2007. Fortunately he continues to participate in the research efforts of the Excellence Cluster "Nanosystems Initiative Munich" (NIM). We congratulate him and wish him continued success.
Another success of our research efforts is reflected in the fact that Alex Holleitner, a Junior Professor at LMU since 2006 accepted an Associate Professor position, also at the Technical University of Munich, in August 2007. Fortunately, we continue to collaborate intensively. In addition two postdoctoral scientist in our group also accepted attractive position abroad with Joel Moser joining the group of Adrian Bachtold in Barcelona in early 2007 and Humboldt fellow Ivan Favero returning to a tenured position in Paris after nearly two years in Munich. The losses mentioned above were at least in part compensated by Eva Weig joining our group on a six year appointment aiming towards the so-called "Habilitation" after spending two years as postdoc in Santa Barbara on realizing superconducting qubits. Back in Munich her research now concentrates on nano-electro-mechanical systems. We also had to master major changes in our technical staff with our engineer Stefan Schöffberger joining a technical company as codirector and our technician Alexander Paul accepting a new job closer to his family. We thank them for their efficient support and also welcome their respective successors, Philipp Altpeter and Reinhold Rath.
In addition to Khaled Karrai, who continues to be a highly desired research partner and guest in our group, as time permits besides his main task as CTO of Attocube we were happy to host visiting scientists from around the world. These included Sasha Govorov (University of Ohio, Athens), Richard Warburton (Heriot Watt University, Edinburgh), and Kenji Ikushima (University of Tokyo) as visiting professors as well as Song Li (Chinese Academy of Sciences, Bejing) as postdoctoral research fellow supported by the Alexander von Humboldt Foundation. In addition our research collaborators Itai Carmeli, Ivan Favero and Vadim Khrapay joined us for shorter visits thus continuing successful international collaborations.
With ten students completing their diploma thesis and five students successfully finishing their doctoral degree 2007 was also a very successful year in our educational efforts. In addition to those research students that now moved with Friedrich Simmel and Alex Holleitner the Technical University Munich in Garching four new diploma students started with their thesis projects and we accepted three new doctoral students. Our scientific activity is also reflected by the publication of 22 papers in international journals and quite a few invited talks at international conferences and institutions. Most importantly, attractive professional positions given to many members of the group at all levels mirror best the success of our research and educational effort.
It is my pleasure to thank all group members for their continued enthusiasm and excellent research and I wish those that have left us in 2007 all the best for their new careers. I also gratefully acknowledge the generous support by our funding agencies. We hope that many readers worldwide enjoy this report and are happy to receive any feedback.
Munich, April 7th, 2008
Jorg P. Kotthaus
Electronic properties
Reducing the length scales of transistors well below 100 nanometers is one of the present key efforts in semiconductor industry. At the same time such tiny structures allow the observation of phenomena obeying the fundamental laws of quantum mechanics. In our clean room we fabricate a variety of gate-controlled devices by e-beam lithography starting from GaAs/AlGaAs heterostructures that contain a two-dimensional electron system. One of our main efforts aims at possible applications in quantum information technology of interacting zero-dimensional quantum dots (QD). In 2007 one of the topics was the back-action of a biased quantum point contact (QPC) onto a device consisting of two or three coupled QDs. This is an important issue, since biased QPCs are commonly used as detectors of the charge configuration of coupled QDs. Energy exchange with the detector causes the quantum states in the coupled QDs to de-cohere and limits the benefit for possible applications in quantum information processing. We demonstrate that the energy exchange can be observed directly in bounded regions of the charge stability diagram of the coupled QDs device. Another source of de-coherence is the ambient temperature in the solid state system. In order to study such influences freely suspended QDs were realized. In these systems the phonon spectrum can be re-engineered allowing for specific investigations of the interaction between phonons and localized electron states in coupled QDs. To read out the result of a quantum calculation high frequency charge detection is desirable. We develop tank-circuits for the high frequency detection of the conductance of a QPC used as charge detector. In this project it is planned to realize spin quantum bits, perform spin to charge conversion and high frequency detection of the charge state.
Freely suspended quantum dots
Clemens Rössler, Martin Herz, Jörg P. Kotthaus, and Stefan Ludwig, in collaboration with Max Bichler, Dieter Schuh, and Werner Wegscheider
Quantum dots embedded in nanoscale phonon cavities are employed in order to investigate electron-phonon interactions. Our phonon cavities are nanoscale bridges excavated from AlGaAs/GaAs heterostructures containing a two-dimensional electron system (2DES). The 2DES can be further structured in order to define one-dimensional-constrictions (quantum point contacts) or zero-dimensional-systems (quantum dots). Until recently, negative voltages applied to metal top gates were used to deplete the 2DES. However, it turned out that in these devices tiny leakage currents between the biased top-gates and the 2DES result in strong telegraph noise. Therefore, a revised sample design now employs etched constrictions combined with in-plane sidegates containing a 2DES (Fig. 1).
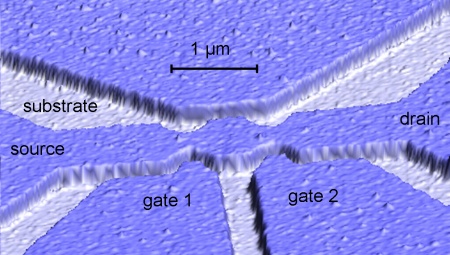
Figure 1.AFM micrograph of a quantum dot device, taken before underetching. Elevated regions inherit active 2DES. Two constrictions define tunnel barriers of a quantum dot. In-plane gates 1 and 2 can be used for further tuning of the tunnel barriers and the quantum dot size.
These devices turn out to be well suited to define stable quantum dots necessary to investigate the influence of cavity phonons onto the quantum dot (Fig. 2).
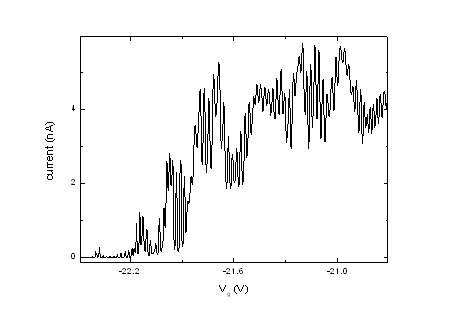
Figure 2. Current through a freely suspended quantum dot as a function of the sum of voltages applied to gates 1 and 2 (see Fig. 1). Each of the 75 small local current maxima marks a change of the quantum dot size by one electron.
In future, we plan to exploit the coupling of discrete mechanical cavity modes to the electronic states of the quantum dot. In addition, we will investigate the influence of the modified phonon spectrum within microscale bridges onto the coherence time of electronic states in quantum dots.
Towards high frequency charge detection using a tank circuit
Dawid Kupidura, Jörg P. Kotthaus, and Stefan Ludwig, in collaboration with Werner Wegscheider
Radio frequency charge detection is necessary for a useful design for semiconductor based quantum information processing. High frequency charge detection will make single shot readout at high rates possible. It would also allow to switch off the detection during qubit rotation in order to avoid decoherence caused by the backaction of the detector. As a first step towards high frequency detection a tank-circuit was designed (Fig. 1). The reflection coefficient of a radio frequency signal strongly depends on the transmission of a quantum point contact (QPC).
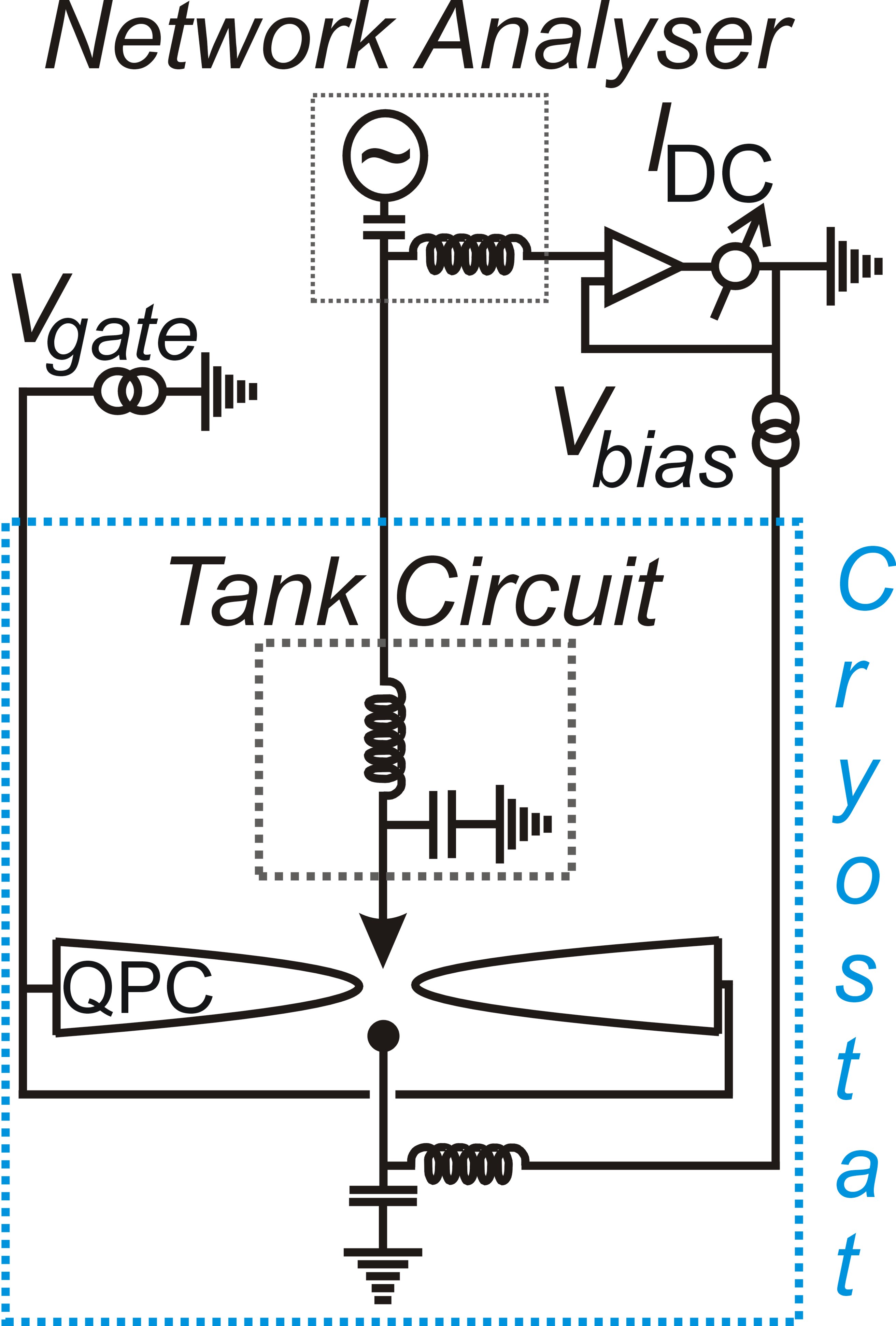
Figure 1. Experimental setup used to measure the rf-reflection on a QPC test device.
Since the QPC transmission is a function of the local electrostatic potential such a tank circuit can be used as a fast detector [1] of the charge states of coupled quantum dot devices [2, 3]. Fig. 2 shows the dc-conduction of QPC (filled black circles) as a function of the voltage applied to the top gates used to shape the QPC in the two-dimensional electron gas 90 nm below the surface. The first two conductance plateaus at G = 2 e2/ h and G = 4 e2/ h can be resolved. Also shown the reflection coefficient of the tank-circuit (red open circles). It resembles the dc-conductance and therefore proofs that our tank-circuit works. The next step will be to include a low temperature amplifier in the circuit to dramatically improve the detector resolution.
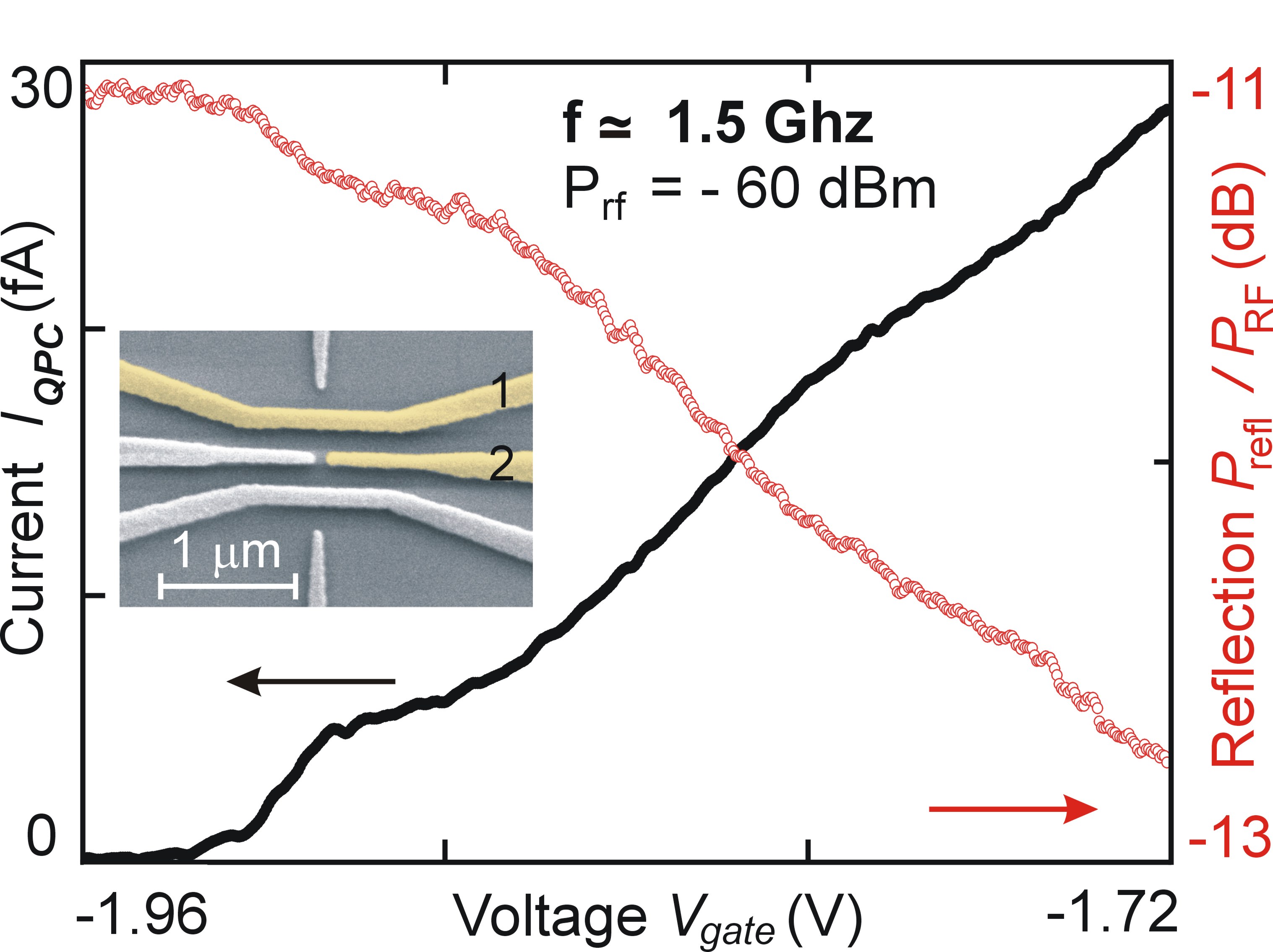
Figure 1. Inset: Scanning electron micrograph of the sample surface containing metal top gates. Yellow gates 1 and 2 are used to laterally define a one-dimensional channel (QPC). Black solid circles plot the dc-current through the QPC measured as a function of the voltage applied to the top gates 1 and 2. Red open circles plot the reflection coefficient of the tank circuit. All features observed in the dc-current are resembled.
- W. Gödel et. al. , Electronics Letters 30, 977-979 (1994).
- R. J. Schoelkopf et. al. , Science 280, 1238-1242 (1998).
- M. C. Cassidy et. al. , APL 91, 222104-1 222104-3, (2007).
Backaction of a quantum point contact detector on coupled quantum dots
Daniela Taubert, Daniel Schröer, Daniel Harbusch, and Stefan Ludwig, in collaboration with M. Pioro-Ladrière (Atsugi-shi , Japan ), A.S. Sachrajda (Ottawa , Canada), Werner Wegscheider (Regensburg), and K. Eberl
Charge detection using a biased quantum point contact (QPC) is a very effective probe for studying few electron quantum dot (QD) circuits. Coupled QDs can also be utilized as qubits. However, the backaction of a detector needed for read out can result in decoherence of the qubit. We investigated the backaction of a biased QPC on coupled QDs in several samples. We find that a biased QPC emits non-equilibrium energy. Absorption of this energy by a coupled triple QD results in telegraph noise where the charge in one QD fluctuates. If these fluctuations are slow enough, they can be observed in a low frequency detection scheme (Fig 1). A similar situation can be reached in a double QD, if only one of the two dots is coupled to the 2DEG (Fig. 2). One of the charging lines ends (arrow) where the tunnel barrier between the left QD and its lead is impenetrable. Here, also a triangular shaped region of telegraph noise indicates the backaction of the biased QPC. Additional experiments show a strong dependence on the bias applied to the QPC [1]. While the telegraph noise can only be observed in bounded regions of the stability diagram because of the finite bandwidth of the detector, we expect telegraph noise at higher frequencies throughout the stability diagram.
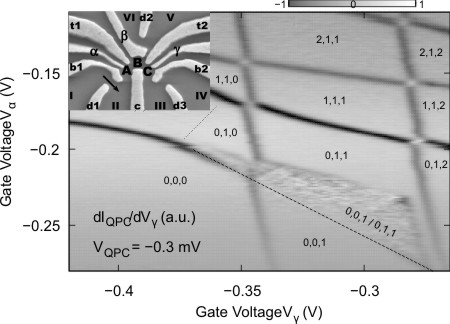
Figure 1. Stability diagram of a serial triple QD (gate layout in the inset) measured with a nearby QPC (arrow in the inset). One can see a triangular shaped region of strong telegraph noise. The numbers indicate the charge configuration in the QDs.
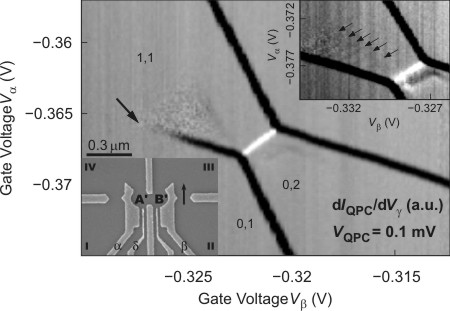
Figure 2. Stability diagram of a double QD (gate layout in the lower inset). Telegraph noise is observed where the charging line fades.
- D. Taubert et al., arXiv:0801.4002 (2008)
In-situ measurements of pentacene thin film transistors during growth
Matthias Fiebig, in collaboration with Daniel Beckmeier, and Bert Nickel
In order to get a better understanding of charge transport in organic transistors, we have performed electronic in-situ measurements at pentacene thin film transistors during pentacene film growth in a high vacuum chamber (Fig.1). Our measurement setup allows us to determine the electronic characteristics of our transistors with a thickness resolution of about 1Å. Source-Drain-Current already starts to flow at a pentacene thickness of about 10Å which is less than a fully completed monolayer (see Fig. 2a). For films beyond a certain threshold thickness (about 50Å for the TFT in Fig. 2b) the transistors behave as expected by standard theory, i.e. constant mobility and hysteresis but linearly increasing threshold voltage. Transistors below this threshold thickness show a strong increase in transconductance and threshold voltage while the hysteresis decreases clearly with increasing film thickness (see Fig. 2b). This could be explained by a model that takes percolation into account. Moreover charge traps not only at the pentacene/dielectric interface but also at the pentacene surface could contribute to the observed thickness dependence.
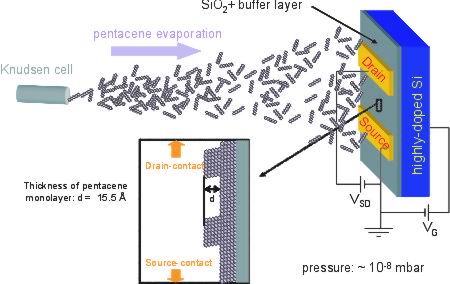
Figure 1. Sketch of the measurement setup. 15.5Å thick monolayers of pentacene molecules grow and hence complete the bottom contact thin film transistor.
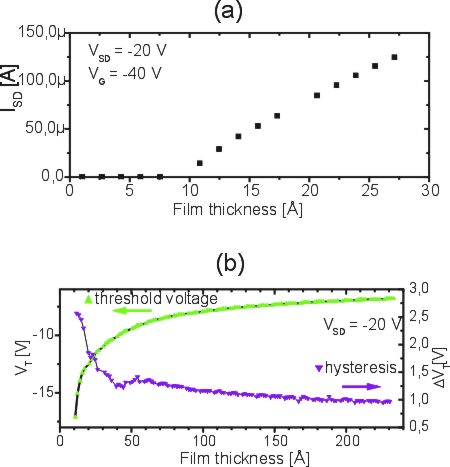
Figure 2. (a) Source-drain-current at a source-drain voltage of VSD = -20 V and a gate voltage of VG = -40 V in dependence of the pentacene film thickness. (b) Threshold voltage and hysteresis in dependence of pentacene thin film thickness determined by using gate sweeps at VSD = -20 V.
Optical and optoelectronical phenomena in nanosystems
The optical manipulation of electronic excitations in nanostructures was at the center of our research also in the year 2007. To this end, optically generated excitons were studied in various solid state environments. A. Gärtner et al. exploited the recently realized electrostatic traps to store long-living excitons in coupled GaAs quantum wells in order to investigate the loading dynamics of excitons in such traps. K.-D. Hof et al. investigated lithographical methods to fabricate submicron channels, which are suitable for optoelectronic experiments on the mesoscopic scale. S. Seidl et al. performed an in-depth analysis of the fine-structure splitting of the excitonic states in self-assembled quantum dots. M. Kroner et al. demonstrated that excitonic states in self-assembled quantum dots can be sufficiently described by a two-level scheme. Both experiments were performed under the guidance of K. Karrai, and they showed the applicability of quantum optical schemes self-assembled quantum dots. A.W. Holleitner et al. described experiments on the dimensionally constrained transition of spin relaxation processes in InGaAs channels. The corresponding article on the transition from two-dimensional to one-dimensional spin dynamics was featured by the “10 Anniversary Highlights” of the New Journal of Physics. The group of A.W. Holleitner found also an efficient way to covalently couple the photosynthetic reaction center PSI to carbon nanotubes. This scheme allows exploiting the potential of photosynthetic proteins as integrated parts of organic nanodevice for optoelectronic applications.
Micropatterned Electrostatic Traps for Indirect Excitons in Coupled GaAs Quantum Wells
Andreas Gärtner, Alexander W. Holleitner, and Jörg P. Kotthaus, in collaboration with Dieter Schuh (Universität Regensburg, Germany)
For detecting the Bose-Einstein condensation of excitons, it is a prerequisite to define controllable confinement potentials for excitons. Andreas Gärtner et al. realized an electrostatic trap for excitons that gives rise to a very steep harmonic trapping potential for indirect excitons in one dimension [1]. The trapping mechanism relies on a local electrostatic field enhancement in combination with the quantum confined Stark effect. The indirect excitons are trapped in GaAs quantum wells just below the perimeter of SiO2 layers, which are sandwiched between the surface of the GaAs heterostructure and a semitransparent metallic top gate. The heterostructures were grown by Dieter Schuh then at the Walter Schottky Institut of Technische Universität München. The authors explain the exciton trapping via the electrostatic influence of surface states at the GaAs/SiO2 interface. The authors find nearly harmonic trapping potentials with spring constants of ~10 keV/cm2. The value exceeds previous results on coupled quantum wells by a factor of 300. Such electrostatic traps for indirect excitons may ultimately be exploited for hosting an excitonic Bose-Einstein condensate. Andreas Gärtner et al. also investigated the loading mechanisms of such traps [2].
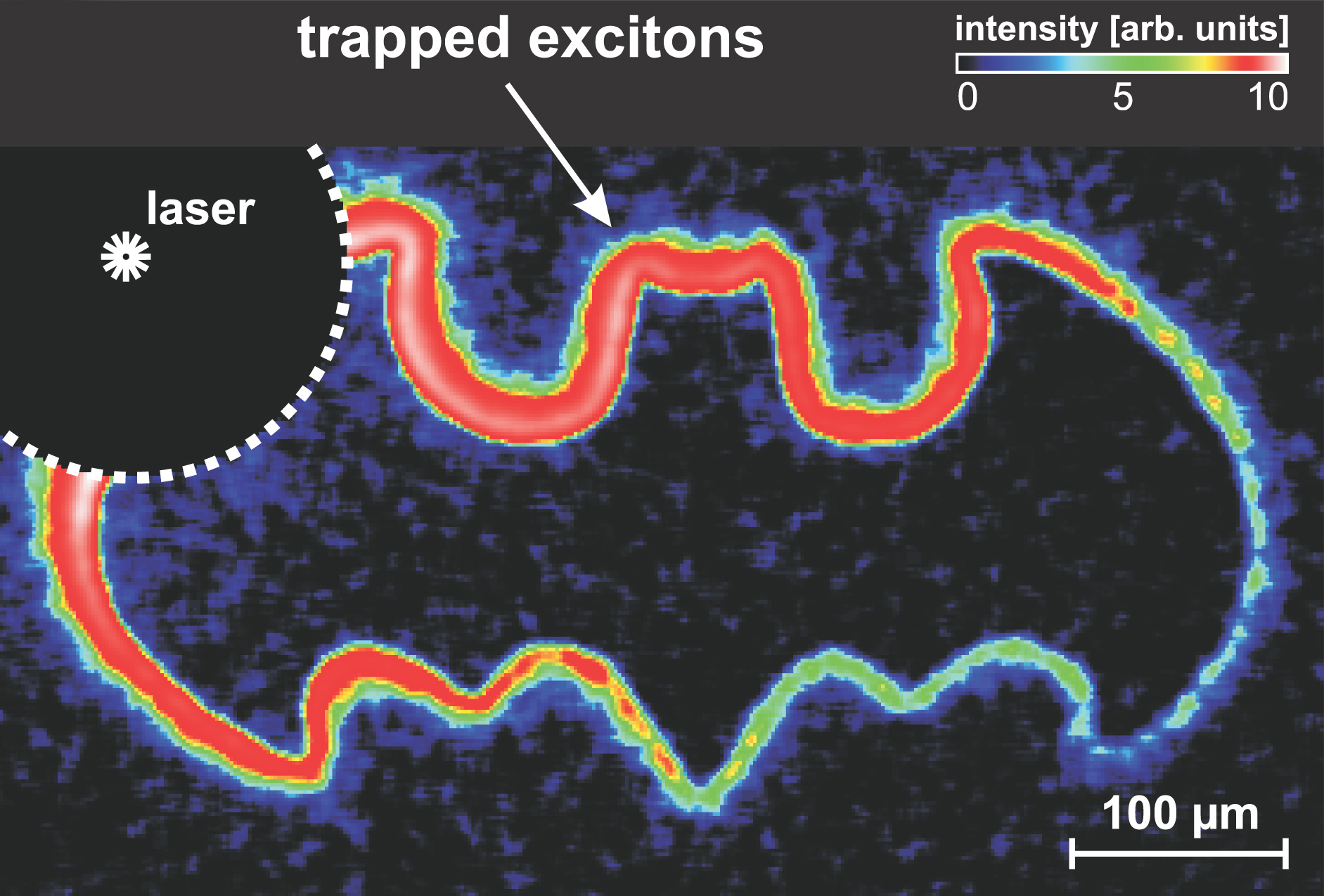
Figure 1. Excitons captured and stored along the perimeter of a SiO2 layer on top of a GaAs/AlGaAs heterostructure. The intensity of the resulting observed photoluminescence is color-coded.
- A. Gärtner, L. Prechtel, D. Schuh, A. W. Holleitner, and J. P. Kotthaus, Phys. Rev. B 76, 085304 (2007).
- A. Gärtner, D. Schuh, A.W. Holleitner, J.P. Kotthaus, Physica E (2007), doi:10.1016/j.physe.2007.10.042
Optically induced charge transport through submicron channels
Klaus-Dieter Hof, Clemens Rössler,Stefan Ludwig, and Alexander W. Holleitner in collaboration with W. Wegscheider (Universität Regensburg, Germany)
Klaus-Dieter Hof et al. investigated the optically induced charge transport through conducting submicron channels in AlGaAs/GaAs heterostructures. The channels were defined within a two-dimensional electron gas in a quantum well by chemical etching (see Figure. 1). The heterostructures were provided by Werner Wegscheider, Universität Regensburg, Germany. By applying a voltage to a top gate, the channels could be depleted electrostatically. In the work Klaus-Dieter Hof et al. exploited different heterostructure designs and processing techniques to build nanostructured optoelectronic detectors [1]. The presented methods demonstrated the suitability of the devices for optically induced charge transport measurements in the mesoscopic regime.
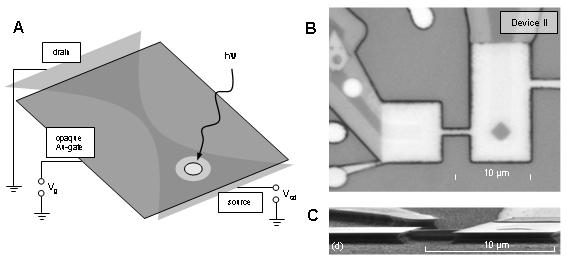
Figure 1. (A) Experimental circuit. A lateral constriction in a two-dimensional electron gas between source and drain contacts forms a low-dimensional electron channel. The central areas of the devices are covered with an opaque top gate (bright rectangle). An aperture in the top gate close to the constriction defines the position where the underlying two-dimensional electron gas is optically excited. (B) SEM top view of a typical device. The opaque top gate covers the central region of the device. Underetched regions appear as pale areas. (C) SEM micrograph of a similar device taken under a tilt angle of 87 degrees.
- Klaus-Dieter Hof, Clemens Rössler, Werner Wegscheider, Stefan Ludwig, Alexander W. Holleitner, Physica E 40 1739–1741 (2008)
Statistics of quantum dot exciton fine structure splittings and their polarization dependence
Stefan Seidl, Martin Kroner, Alexander Högele, Alexander W. Holleitner, and Khaled Karrai in collaboration with B.D. Gerardot, P.A. Dalgarno, K. Kowalik, R.J. Warburton (Heriot-Watt University, Edinburgh, UK) and J.M. Garcia, P.M. Petroff (University of California, Santa Barbara, USA)
Self-assembled semiconductor quantum dots can be considered as artificial atoms. In contrast to real atoms quantum dots have the advantage of being embedded in a host material so that no intricate traps are needed to study a single one [1]. However, the solid-state host material can also introduce deviations from perfect symmetry. The lack of symmetry in the quantum dot lifts the natural degeneracy of the ground state, creating the fine structure splitting. Elimination of the fine structure splitting allows for the generation of entangled photon pairs from the biexciton to exciton to vacuum state cascade. Therefore, a detailed characterization of the fine structure splitting is essential. Stefan Seidl et al. investigated the fine structure splitting of several tens of single quantum dots, which were grown in the laboratory of Pierre Petroff at the University of California, Santa Barbara, USA, using the partially capped island method [2]. In particular they found a large variation in the fine structure splitting, which is independent of emission wavelength, for such quantum dots (see Figure 1B). S. Seidl et al. observed further that quantum dots with a fine structure splitting below 30 μeV do not show a dipole alignment along the crystal axis, as it is widely believed. Hence, there is always the availability of a quantum dot with small fine structure splitting and suitable orientation to choose for active quantum optical manipulation.
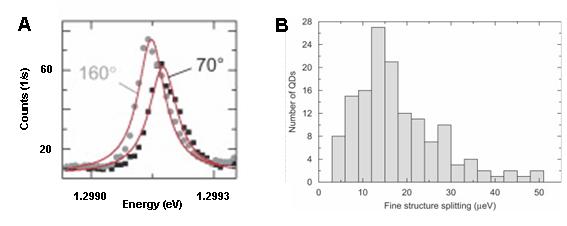
Figure 1. (A) Two photoluminescence spectra of a single quantum dot recorded for linear polarizations of 70 and 160 degree relative to the [110] direction of the GaAs substrate. The drawn lines are Lorentzian fits, by which the fine structure splitting can be deduced [2]. (B) Histogram of the fine structure splitting of 142 quantum dots, showing a broad distribution.
- S. Seidl, A. Högele, M. Kroner, K. Karrai, R. J. Warburton, J. M. Garcia, and P. M. Petroff, phys. stat. sol. (a) 204, No. 2, 381–389 / DOI 10.1002/pssa.200673956 (2007).
- S. Seidl, B.D. Gerardot, P.A. Dalgarno, K. Kowalik, A.W. Holleitner, P.M. Petroff, K. Karrai, R.J. Warburton, Physica E, doi:10.1016/j.physe.2007.10.046 (2007).
Rabi splitting and ac-Stark shift of a charged exciton
Martin Kroner, Christine Lux, Stefan Seidl, Alexander W. Holleitner, and Khaled Karrai in collaboration with A. Badolato, P. M. Petroff, and R. J. Warburton
The Rabi splitting of the negatively charged exciton in a single InGaAs quantum dot is observed in resonance transmission spectroscopy. We use a pump laser excitation to drive strongly the unpolarized trion transition in a quantum dot and detect its modified absorption spectrum with a second weak probe laser. The observed Rabi-splitting of the trion resonance line is shown in Fig 1 (a) for different pump laser powers. By tuning the pump laser near resonance, we observe an ac-Stark effect dispersion as shown in Fig. 1 (b). Both observed effects are signatures of a strongly coupled two level system. We combine the results of pump probe with saturation spectroscopy data to deduce the contributions of the decay and decoherence rates as well as spectral fluctuations to the low power linewidth. [1]
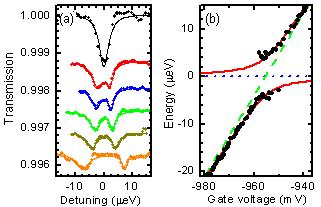
Figure 1. (a) Differential transmission spectra of the negatively charged exciton transition in a single quantum dot, without pump laser (top curve in black) and with increasing pump laser power (below) up to 450nW (orange). (b) Energy shift of the resonance as a function of the detuning of the pump laser energy (via the gate volatage) from the resonance condition. A clear anti-crossing with the pump laser energy (set to zero) is observed.
- M. Kroner, et al., APL 92, 031108 (2008).
Optical detection of single electron spin resonance in a quantum dot
Martin Kroner, Kathrina M. Weiß, Benjamin Bidermann, Stefan Seidl, Stefan Manus, Alexander W. Holleitner, and Khaled Karrai in collaboration with A. Badolato, P. M. Petroff, Brian D. Gerardot, and R. J. Warburton
We demonstrate optically detected spin resonance of a single electron confined to a self-assembled quantum dot. The dot is rendered dark by resonant optical pumping of the spin with a coherent laser (Fig. 1 (a)). The contrast is restored by applying a radio frequency (rf) magnetic field at the spin resonance. The scheme is sensitive even to rf fields of just a few μT that can be applied to the quantum dot by a simple loop antenna as depicted in Fig. 1 (b). The narrow electron-spin resonance line (Fig. 1 (c)) makes the determination of the electron spin splitting - given by the electron g-Factor - a crucial prerequisite for the experiment. We developed a two-laser resonant spectroscopy scheme to optically determine directly the electron Zeeman splitting with high precision (see Fig. 1 (a)). [1]
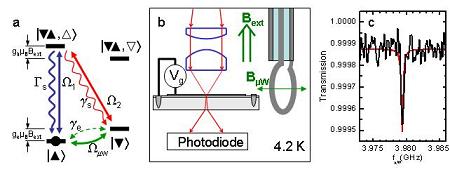
Figure 1. (a) Level-schema of the negatively charged exciton in a magnetic field. The ground and excited states are split by the Zeeman energy of the electron and hole respectively. A resonant laser (blue arrow) pumps the electron spin via a Raman transition (red curved arrow) into to the spin-down ground state. A resonant microwave field (green arrow) restores the equal population of the ground states and thereby allows again the absorption of the pump laser (blue arrow). A second laser (red arrow) is used to probe the Raman transition allowing a direct measurement of the Zeeman splitting of the electron ground state. (b) Schematic drawing of the confocal microscope objective to illuminate the sample. The photodiode measures the transmitted laser light. The microwave field is provided by a single loop antenna generating an oscillating magnetic field perpendicular to the external magnetic field. The whole setup is immersed in a bath cryostat and operates at 4.2 K. (c) Tranmission signal of the pump laser (blue arrow in (a)) as a function of the microwave frequency. The absorption of the laser is reestablished in case of resonance leading to a narrow dip in the transmission spectrum.
- M. Kroner, et al., accepted for publication in PRL (2008).
The Nonlinear Fano Effect
Martin Kroner, Sebastian Remi, Benjamin Biedermann, Stefan Seidl, and Khaled Karrai in collaboration with A. O. Govorov, W. Zhang, A. Badolato, P. M. Petroff, B. D. Gerardot, R. Barbour, and R. J. Warburton
The Fano effect is very well known from spectroscopy on atoms, since it was explained by U. Fano in 1961 [1]. It arises when quantum interference takes place between two competing optical pathways, one connecting the energy ground state and an excited discrete state, the other connecting the ground state with a continuum of energy states. The nature of the interference changes rapidly as a function of energy, giving rise to characteristically asymmetric lineshapes. Whereas Fano’s original theory applies to the linear regime at low power, at higher power a laser field strongly admixes the states and the physics becomes rich, leading, for example, to a remarkable interplay of coherent nonlinear transitions. We report experiments that access the nonlinear Fano regime by using semiconductor quantum dots, which allow both the continuum states to be engineered and the energies, that lie originally in the deep ultraviolet, to be rescaled to the nearinfrared. In Fig.1 differential transmisison spectra of a quantum dot for different laser excitation powers are shown (c-h). The quantum dot was weakly coupled to a continuum of states and the characteristic Fano shape of the resonance gets more pronounced with increasing excitation power. We developed a general theory that explaines the non-linear Fano effect and the corresponding, calculated specta are shown in Fig.1 (i-n). [2]
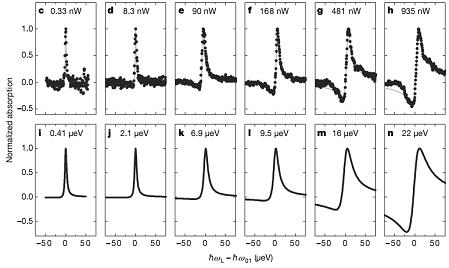
Figure 1.Differential tranmsission spectra of the non-linear Fano effetc observed on a singel quantum dot, weakly coupled to a continuum of states (c-h). The corresponding calculated spectra are shown in (i-n). The excitation power and corresponding Rabi energy is given respectively.
- U. Fano, Phys. Rev. 124, 1866–1878 (1961).
- M. Kroner, A. O. Govorov, et al., Nature 451, 311 (2008).
Dimensionally constrained Dyakonov Perel spin relaxation in n-InGaAs channels: transition from 2D to 1D
Alexander W. Holleitner, in collaboration with Vanessa Sih, R.C. Myers, A.C. Gossard, D.D. Awschalom (University of California, Santa Barbara, USA)
In the emerging field of spintronics, it is important to explore carrier spin relaxation mechanisms in nanostructures as a function of dimensionality. In two and three dimensions, elementary rotations do not commute, with significant impact on the spin dynamics if the spin precession is induced by spin-orbit coupling. Spin-orbit coupling creates a randomizing momentum-dependent effective magnetic field; the corresponding relaxation process is known as the D’yakonov-Perel’ mechanism. In an ideal one-dimensional system, however, all spin rotations are limited to a single axis, and the D’yakonov-Perel’ spin relaxation is suppressed. The authors demonstrated a progressive slowing of the D’yakonov-Perel’ spin relaxation in the regime approaching the one-dimensional limit [1]. The experiments were performed at the University of California, Santa Barbara in USA, on narrow channels of semiconductor heterostructures, containing InGaAs quantum wells, in which the spin-orbit interactions are dominated by structural inversion asymmetry [2,3]. Such solid-state systems have been proposed as candidates for spintronic devices, including spin transistors, due to their potential scalability and compatibility with existing semiconductor technology.
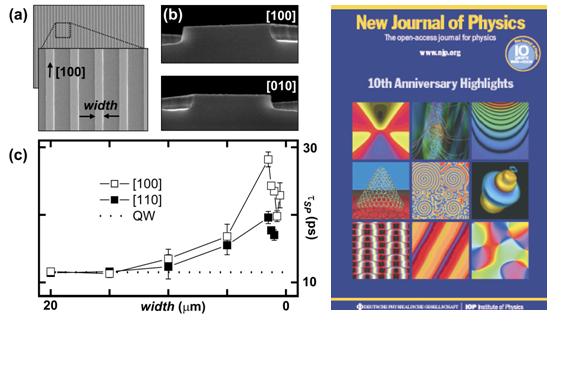
Figure 1. (a) Scanning electron micrograph of dry-etched InGaAs channels, which are patterned along the four crystallographic directions [100], [110], [010] and [-110]. (b) Micrographs of channels along the cleaving direction [110]. The crystallographic direction of the channels is [100] and [010]. (c) Spin relaxation time τSP as a function of the channel width. Open and filled squares represent data of channels along [100] and [110], while the dotted line depicts the spin relaxation time of the unstructured quantum well.
- A. W. Holleitner, V. Sih, R. C. Myers, A. C. Gossard, D. D. Awschalom, Physical Review Letters 97, 036805 (2006).
- A. W. Holleitner, V. Sih, R. C. Myers, A. C. Gossard, D. D. Awschalom, New Journal of Physics 9, 342 (2007).
- See also the corresponding feature article in the 10th anniversary of New Journal of Physics at http://www.iop.org/EJ/journal/-page=extra.top5/1367-2630
A photosynthetic reaction center covalently bound to carbon nanotubes for nanoscale optoelectronic circuits
Simone Lingitz, Bernd Zebli, Markus Mangold, Alexander W. Holleitner in collaboration with I. Carmeli, C. Carmeli, S. Richter (Tel Aviv University, Israel)
The authors covalently bound the Photosystem I (PS I) to carbon nanotubes (CNTs). The PS I is a protein complex located in the thylacoid membrane of plants, algae and cyanobacteria which mediates the light-induced electron transfer in the photosynthetic pathway. As a nano-sized, high-efficient bioenergetic unit, the photosynthetic reaction centre is a promising candidate for applications in molecular nano-optoelectronics [1]. In order to electrically contact the photoactive proteins, a cysteine mutant is generated at one end of the PS I by genetic engineering (in the groups of I. Carmeli and C. Carmeli at Tel Aviv University, Israel) and to this reactive group the CNTs are covalently bound via chemical self-assembly using carbodiimide chemistry. Due to this combination of an energy transformation and a transport unit, this hybrid nanosystem provides an ideal basis for optoelectronic applications.
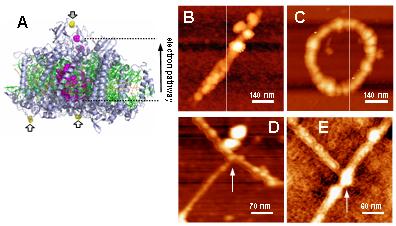
Figure 1. (A) Molecular structure image of the photosynthetic reaction center I (PSI) based upon crystallographic data. The PSI protein has a cylindrical shape with a diameter of about 15 nm and a height of 9 nm, and it is composed of polypeptide chains (gray) in which chlorophyll (green) and carotenoids (orange) are imbedded. The black arrow schematically depicts the light induced charge separation across the PSI, which starts at the special chlorophyll pair P700. The chromophores which mediate the electron transfer are represented by the space fill model (cyano). The PSI covalently binds to chemically functionalized carbon nanotubes through Cys mutations along the polypeptide backbone (arrows and space fill model, yellow). (B) and (C) Atomic force micrographs of PSI bound to carbon nanotubes. The images show a large number of PSI with a diameter of about 10-20 nm bound to the side-walls and the tips of CNTs. (D) and (E) AFM images taken of CNT Ö PSI Ö CNT hybrid junctions.
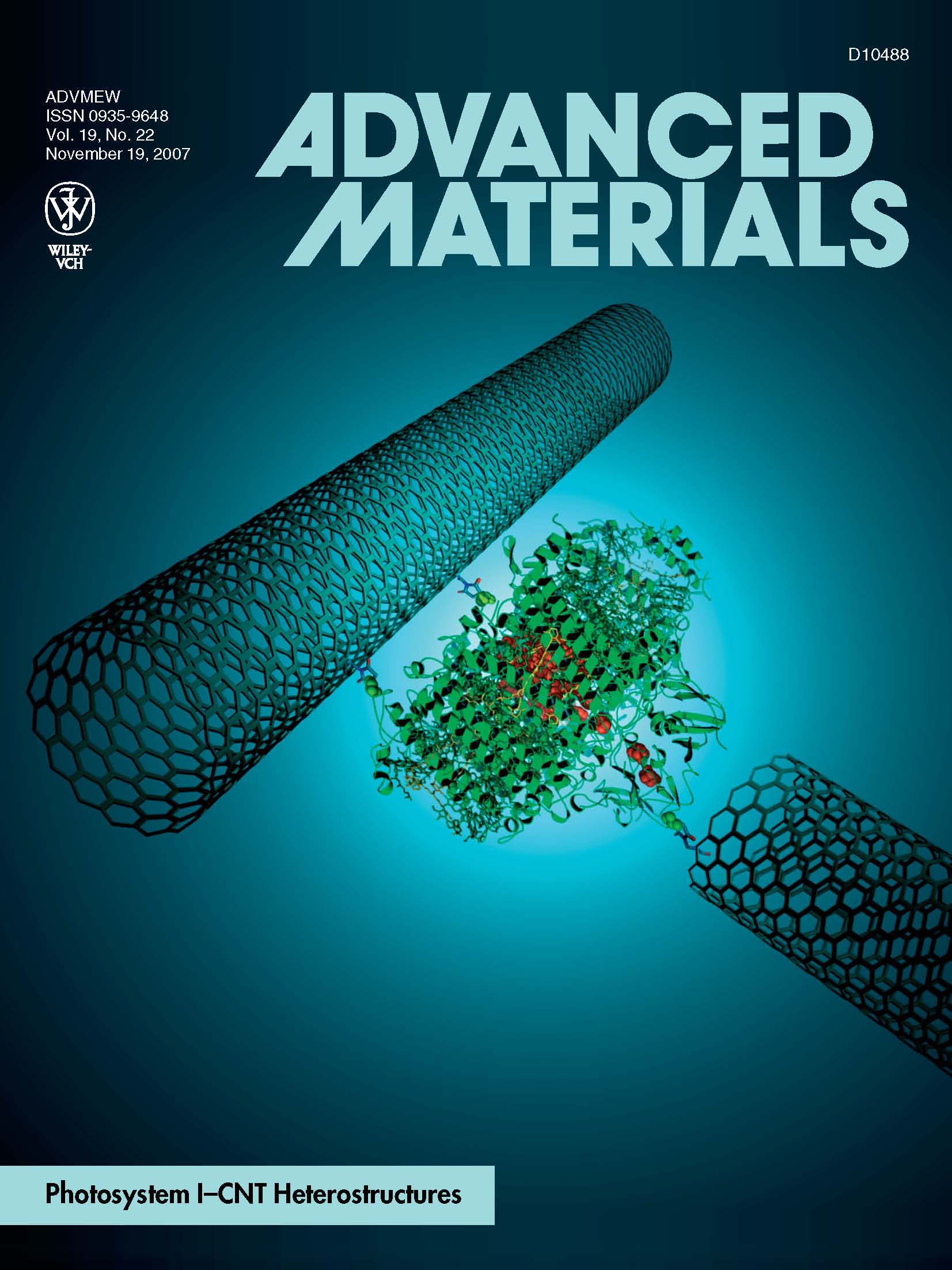
- I. Carmeli, M. Mangold, L. Frolov, B. Zebli, C. Carmeli, S. Richter, and A.W. Holleitner, Advanced Materials 19, 3901 (2007).
Nanomechanical systems
Our research on nanomechanical systems is centered around the investigation of the fundamental physical properties of mechanical resonators at the nanoscale. Those resonators are realized by lithographic techniques capable of creating free-standing objects with thickness and lateral dimensions down to about some 10 nanometers. A fascinating aspect of this field is that further insight can be gained by combining it with other fields such as electronics or optics. For example, capacitive coupling in nanoelectromechanical devices allowed us to investigate the mechanically actuated current across a nanomechanical charge shuttle. Optomechanical coupling has been employed to study the interaction between photons stored in a high finesse Fabry-Perot cavity and a mechanical nanoresonator, whereas coupling a nanomechanical resonator to the atoms of a Bose-Einstein condensate allows to build a bridge to atomic physics. In order to optimize our resonators for those projects, as well as for applications as sensitive sensors and actuators, a detailed understanding of their actuation and control as well as their physical properties such as resonance frequency or dissipation is required. Progress on the actuation and characterization of doubly clamped as well as singly-clamped nanomechanical resonators is also reported.
Nano-mechanical electron transport at 20 Kelvin
Daniel R. König, Eva M. Weig, and Jörg P. Kotthaus
We have demonstrated operation of a nano-mechanical single electron shuttle (MSET) at 20 Kelvin, which represents a major step towards the realization of one-by-one electron transport in the Coulomb blockade regime. The MSET we fabricate consist of a freely suspended silicon nitride string under a tensile stress of 1.38 GPa. The string is 1400 nm in length, 70 nm in width, and 100 nm in height. A gold island with dimensions of about 140 nm in length, 170 nm in width, and 60 nm in height is located at the center of the string. The silicon nitride string is excited by ultrasonic waves via a piezo actuator, which drives the mechanical vibration without inducing electrical crosstalk. The gold island oscillates and thus shuttles electrons between the source and the drain electrodes which are placed within a distance of 80 nm to either side of the island (Fig. 1). The gold structures located symmetrically on the string to the left and right of the island in Fig. 1 are weights to tune the eigenfrequency of the MSET to a value accessible by the piezo driving system. Figure 2 shows the time-averaged source-drain current Isd for one MSET as function of the driving frequency, clearly displaying a peak at the mechanical resonance frequency of the device.
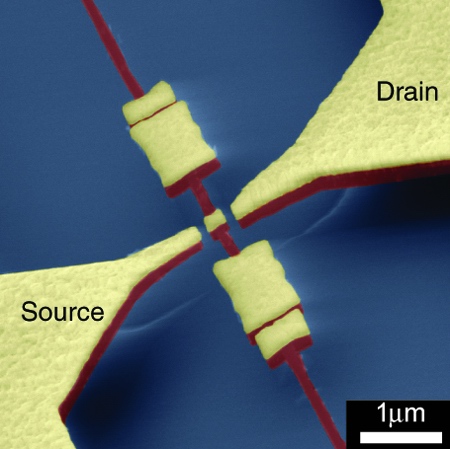
Figure 1. A false-color scanning electron micrograph taken at an angle to reveal the 3D character of the MSET. A gold (yellow) island is located at the center of a doubly clamped freely suspended silicon nitride (red) string. The gold island can shuttle electrons between the source and drain electrode when excited by ultrasonic waves.
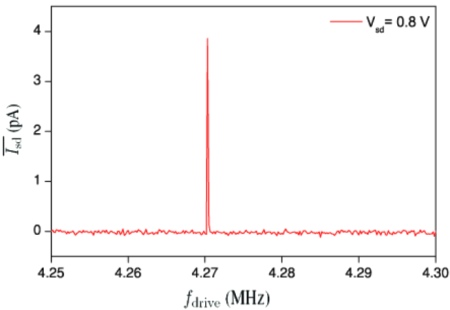
Figure 2. a) Time-averaged current Isd measured between source and drain as function of the driving frequency for a driving power of 17 dBm and a source-drain-voltage Vsd of 0.8 V. The data was taken at a temperature of about 20 K and an exchange gas pressure of 7.5 x 10-4 mbar.
An optomechanical system at the nanoscale
Ivan Favero, Sebastian Stapfner, Phillip Paulitschke, Heribert Lorenz, Eva M. Weig, Khaled Karrai in collaboration with David Hunger, Jakob Reichel, Theodor W. Hänsch
We investigated the interaction between photons stored in a high finesse Fabry-Perot cavity of small mode volume and the vibrational motion of a mechanical nanoresonator placed into the cavity mode as seen in Fig. 1. To this end, we have employed a fiber-integrated optical micro-cavity with a mode waist in the range of only 5 micrometers that was realized in collaboration with the Hänsch group. Into the cavity, we introduced an electron-beam-deposited nanorod of about 4 microns length as a nanomechanical resonator. Rods as the one displayed in Fig. 2 are grown at the very end of a commercial AFM lever by the spinoff Nanotools. The nanorod is introduced and aligned within the cavity mode using a nanopositioning system by the spinoff Attocube. When the nanorod vibrates in the cavity mode, it modulates the cavity transmission, enabling an efficient optical read-out of its vibrational motion. This has allowed us to optically monitor the Brownian motion of the nanorod. This method opens the way to the optical investigation of nanomechanical systems of various sizes, shapes and composition. It also represents a first step towards the study of optomechanical phenomena at the nanoscale, in which light and nanoscopic mechanical degrees of freedom strongly interact. To that respect, an attractive goal of the project is the optical cavity cooling of a nanomechanical resonator to its vibrational quantum ground state [1].
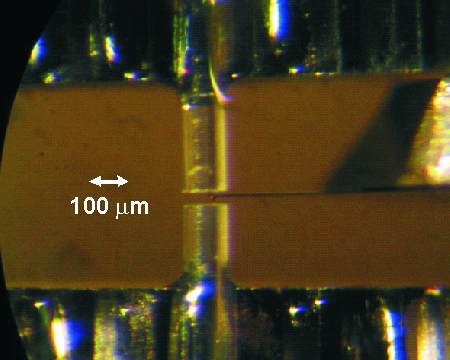
Figure 1. High-finesse optomechanical cavity with nanorod positioned into the cavity mode.
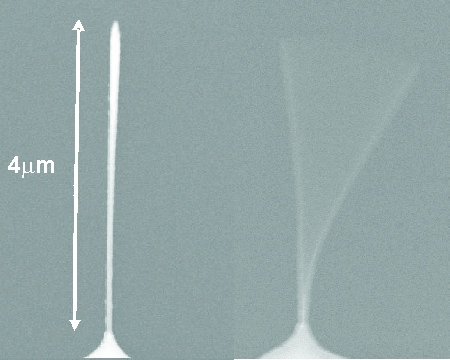
Figure 2. Left: SEM image of 4 micron long electron-beam deposited nanorod used as a mechanical resonator. Right: Vibrating rod under resonant actuation.
- Favero I., Karrai K., arXiv:0707.3117 (2007).
Bose-Einstein condensates coupled to micro- and nanomechanical resonators on an atom chip
In collaboration: David Hunger, Stephan Camerer, Theodor W. Hänsch, and Philipp Treutlein (LMU Munich and Max-Planck-Institute of Quantum Optics), Daniel R. König and Jörg P. Kotthaus (CeNS, LMU Munich), Jakob Reichel (Laboratoire Kastler-Brossel, ENS Paris).
The goal of this collaboration is to couple gaseous atomic Bose-Einstein condensates (BECs) on an atom chip to the mechanical oscillations of micro- and nanomechanical cantilevers. In such a system, the atoms could be used as a sensitive probe and a coherent actuator for the cantilever dynamics. An experiment has been set up in which the motion of magnetically trapped atoms can be coupled to an AFM cantilever (Fig. 1). The coupling is caused by the attractive Casimir-Polder potential experienced by the atoms at sub-micrometer distance from the cantilever surface. In addition, a fabrication process has been developed which allows us to fabricate Co nanomagnets on the tip of nanoscale SiN cantilevers (Fig. 2). The vibrations of such a cantilever with a magnetic tip could be coupled to the spin of the atoms, as theoretically investigated in [1].
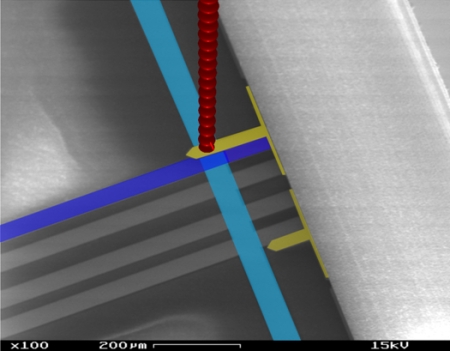
Figure 1.SEM picture of two AFM cantilevers mounted above wires on an atom chip. An auxiliary laser beam can be used for cantilever readout.
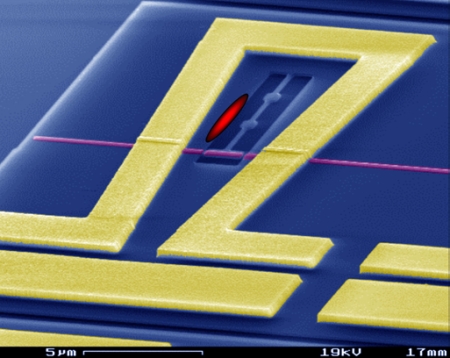
Figure 2.SEM picture of a chip prototype for the magnetic coupling of a BEC to a nanomechanical cantilever with a magnetic tip. Extension of the BEC wave function shown in red for illustration purpose.
- Treutlein P. et al., Phys. Rev. Lett. 99, 140403 (2007).
Nanomechanical resonators under high tensile stress
Quirin Unterreithmeier, Daniel R. König, Eva M. Weig, and Jörg P. Kotthaus
We investigate the mechanical properties of doubly clamped nanomechanical resonators, that have been fabricated from a high stress silicon nitride film on a silicon wafer. Both beam-shaped resonators, as well as resonators hosting a central paddle like the one depicted in Fig. 1 have been fabricated and characterized. Typical dimensions of the doubly-clamped beams are 200 nm x 100 nm x 20 µm in width, height and length, respectively (see Fig. 1). Because of the large intrinsic tensile stress of the suspended material of 1.38 GPa, the resonant motion is only weakly damped, giving rise to high mechanical quality factors, as initially observed by [1]. The resonators are mechanically excited using an acoustic inertial drive mediated via a piezo transducer. The vibration of the resonators has been read out optically with an improved interferometric setup. Figure 2 shows a typical mechanical response of a resonator with a resonance frequency of 7.6545 MHz. Quality factors up to 50,000 are reproducibly achieved at room temperature under vacuum. Under the application of additional external stress, the eigenfrequency can be shifted up to 1%, which corresponds to ~50 FWHM (full width at half maximum).
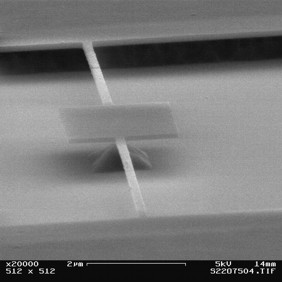
Figure 1.Scanning electron micrograph of a doubly-clamped nanomecanical resonator with central paddle.
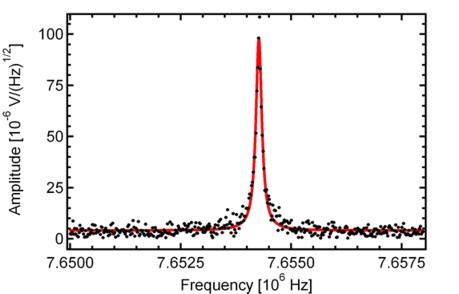
Figure 2.Mechanical response of a nanomechanical resonator with eigenfrequency of 7.6545 MHz and a quality factor of 50,000 measured at room temperature under vacuum.
- Verbridge S. et al., J. Appl. Phys. 99, 124304 (2006)
Statistical analysis of arrays of pillar resonators
Philipp Paulitschke, Heribert Lorenz, Eva M. Weig, and Jörg P. Kotthaus
We succeeded in fabricating large arrays of micron-sized pillars by electron beam lithography followed by reactive ion etching (Fig. 1). The samples are mechanically excited via a piezo transducer while being monitored with the scanning electron microscope in situ. Under resonant actuation the resulting image displays the envelope of the mechanical mode of the pillar, which allows the investigation of the mechanical properties of the individual pillars of the array. The high aspect ratio pillars show eigenfrequencies of the first transverse harmonic mode around 1.5 MHz. The spread of pillar eigenfrequencies of 50 kHz can be fully attributed to geometrical deviations from the anticipated pillar shape (Fig. 2). While lithographic and etching imperfections amount to a lateral error of about 5 nn, a reduced etching rate in the center of the array accounts for a 100 nm variation of pillar height, as could be confirmed by confocal microscopy.
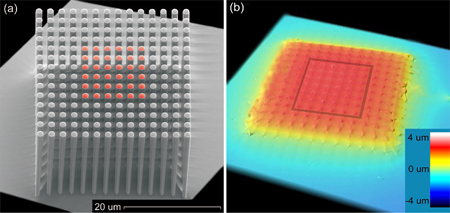
Figure 1. (a) Scanning electron micrograph of a pillar array. The central 36 pillars indicated in red have been investigated for the statistical analysis of mechanical eigenfrequencies. (b) Confocal image of the substrate underlying the array showing the reduced etching depth in the center of the array.
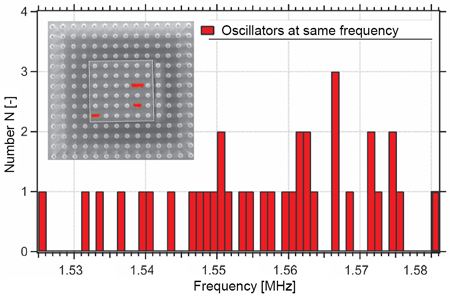
Figure 2. Histogram of the resonance frequencies determined for the first transverse eigenmodes of the central 36 pillars in the array shown in Fig. 1(a). The distribution of eigenfrequencies is mainly due to the variation of pillar length apparent from Fig. 1(b).
A suspended single-walled carbon nanotube resonator
Li Song, Eva M. Weig, Alexander W. Holleitner and Jörg P. Kotthaus in collaboration with Huihong Qian, Achim Hartschuh
Due to its unique strength and low density, carbon nanotubes are attractive and ideal candidates for NEMS structures. By using a floating chemical vapor deposition method, we successfully fabricated suspended single-walled carbon nanotubes under the assistance of an electric field (Fig. 1). To study the mechanical properties of a suspended nanotube resonator, a piezoelectric actuation was used to excite, and an in-situ SEM was performed to detect the vibration of the suspended nanotubes. By sweeping the piezo-actuating frequency, we directly observed the vibration of suspended nanotubes (Fig. 2). As a next step, we plan to investigate transport phenomena under the condition of resonant mechanical and possibly optical excitation performed on the suspended nanotube system.
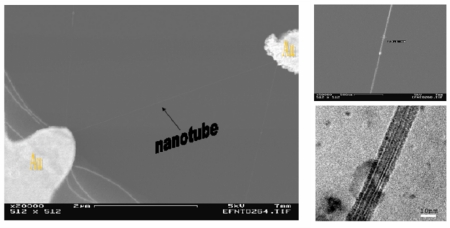
Figure 1.Typical SEM and TEM images of suspended single-walled carbon nanotubes.
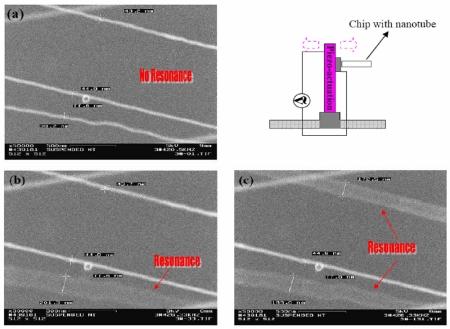
Figure 2.Typical SEM micrographs showing the mechanical resonant behaviors of suspended nanotubes.
Nanobioscience
Determination of DNA melting temperatures in a microfluidic chip
Tim Liedl, and Friedrich C. Simmel
Microfluidic systems offer the possibility to handle and analyze minute amounts of liquid samples. We developed a microfluidic chip for the fast and reliable analysis of DNA melting temperatures in gradients of the denaturing agent formamide [1]. Since formamide lowers the melting temperature of DNA in a linear fashion, a virtual temperature can be ascribed to each position along the gradient. Differences in length of complementary sequences of one nucleotide as well as single nucleotide mismatches can be detected with this method while each measurement is executed in less than one minute.
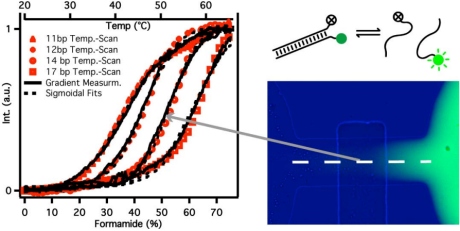
- T. Liedl, F. C. Simmel, Anal. Chem. 79, 5212-5216 (2007)
Trapping and release of fluorescent nanocrystals mediated by signaling DNA molecules
Tim Liedl, and Friedrich C. Simmel in collaboration with H. Dietz, and B. Yurke
The development of stimuli-sensitive drug carriers with programmable structure and properties is of great interest for biomedical applications. The biocompatibility and its versatility as structural material predestine DNA as a building block for such sophisticated carrier systems. We investigated the diffusion properties of fluorescent nanocrystals inside a DNA crosslinked polyacrylamide hydrogel. We were further able to demonstrate, that such gels are capable of trapping and releasing nanoparticles on demand [1]. Due to the biocompatibility of the polymerized polyacrylamide and the crosslinking DNA strands, such gels could find application in the context of controlled drug delivery, where the release of a drug-carrying nanoparticle could be triggered by naturally occurring, potentially disease-related DNA or RNA strands.
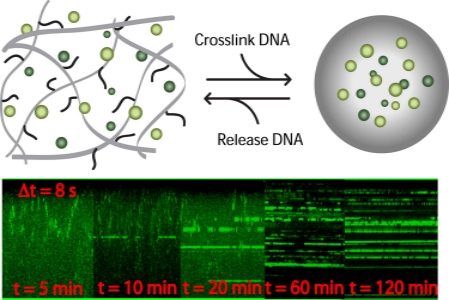
- T. Liedl, H. Dietz, B. Yurke, and F. C. Simmel, Small. 3, 1662-1666 (2007)
Diploma theses completed in 2007
- Benjamin Biedermann
"Optische Spininitialisierung und spinabhängige Laserspektroskopie an einzelnen selbstorganisierten Quantenpunkten" - Lisa Kreuzer
"Photoluminescence of Single Core-Shell Nanowires" - Daniel Harbusch
"Quantenmechanisches Schalten mit ballistischen Elektronen" - Philipp Paulitschke
"Anregung dreidimensionaler Halbleiter Nano-/Mikrostrukturen" - Quirin Unterreithmeier
"Charakterisierung nanomechanischer Resonatoren unter Zugspannung" - Stephan Renner
"Bioelektronische Experimente an Vesikeln, Lipidmembranen und Nanoporen" - Martin Herz
"Elektronentransport durch freitragende Nanostrukturen in GaAs" - Kathrina Weiß
"Kohärente Laserspektroskopie an einzelnen ladungsdurchstimmbaren Quantenpunkten" - Leonhard Prechtel
"Zeitaufgelöste Photostrommessungen an Wellenleiterschaltkreisen" - Georg Schinner
"Heiße Elektronen- und Photonenspektroskopie in zweidimensionalen Strukturen"
PhD theses completed in 2007
- Daniel Schröer
"Tunnelgekoppelte Doppel- und Tripelquantenpunkte auf GaAs im Bereich niedriger Besetzungszahlen" - Andreas Reuter
"DNA-basierte molekulare Maschinen und Aktuatoren" - Tim Liedl
"Towards autonomous DNA-based Nanodevices" - Stefan Seidl
"Resonant interband transmission spectroscopy on single charge-tunable quantum dots" - Constanze Metzger
"Opto-mechanics of deformable Farby-Pérot cavities"
Guest scientists in 2007
- Prof. Khaled Karrai,
attocube systems, München - Prof. Richard Warburton,
Department of Physics, Heriot-Watt University, Edinburgh, UK, SFB 631 - Dr. Vadim Khrapay,
Russian Academy of Sciences, Chernogolovka, Russia, Alexander von Humboldt Stiftung - Prof. Alexander O. Govorov,
Ohio University, "Bessel award" der Alexander von Humboldt Stiftung - Dr. Ivan Favero,
Ecole Normale Supèrieure, Paris Cedex, France, Alexander von Humboldt Stiftung - Dr. Itai Carmeli,
Faculty of Exact Science, Tel Aviv University, Israel, SFB 486 TP A1 - Dr. Kenji Ikushima,
Department of Basic Science, University of Tokyo, Japan - Dr. Song Li,
Chinese Academy of Science, Beijng, China, Alexander von Humboldt Stiftung
Group members in 2007
Senior scientists
- Prof. Jörg P. Kotthaus
- Prof. Alexander Holleitner (until July 2007)
- Dr. Heribert Lorenz
- Dr. Stefan Ludwig
- Dr. Friedrich Simmel (until Octobber 2007)
- Dr. Eva-Maria Weig (since April 2007)
Secretary
- Martina Jüttner
Technical staff
- Philipp Altpeter (since June 2007)
- Hans-Peter Kiermaier
- Wolfgang Kurpas
- Stephan Manus
- Alexander Paul (until July 2007)
- Reinhold Rath (since November 2007)
- Stefan Schöffberger (until July 2007)
Ph.D. students
- Christian Dupraz
- Matthias Fiebig
- Eike Friedrichs
- Andreas Gärtner
- Daniel Harbusch
- Ralf Jungmann
- Constanze Metzger (nee Höhberger)
- Klaus-Dieter Hof
- Daniel König
- Martin Kroner
- Dawid Kupidura
- Tim Liedl
- Simone Lingitz
- Michael Olapinski
- Philip Paulitschke
- Andreas Reuter
- Clemens Rössler
- Stefan Seidl
- Thomas Sobey
- Daniel Schröer
- Quirin Unterreithmeier
- Xaver Patrick Vögele
- Bernd Zebli
Diploma students
- Benjamin Biedermann
- Andreas Fischer
- Bernhard Heindl
- Martin Herz
- José Horas
- Lisa Kreuzer
- Christine Lux
- Michael Müller
- Philip Paulitschke
- Leonhard Prechtel
- Stephan Renner
- Julian Schauseil
- Georg Schinner
- Stefan Schwalb
- Daniela Taubert
- Quirin Unterreithmeier
- Kathrina Weiß
Publications in 2007
- B. D. Gerardot, S. Seidl, P. A. Dalgarno,
R. J. Warburton, D. Granados, J. M. Garcia, K. Kowalik,
O. Krebs, K. Karrai, A. Badolato, P. M. Petroff
“Manipulating exciton fine structure in quantum dots with a lateral electric field“
Appl. Phys. Lett. 90, 041101 -1 – -3 (2007) - F. W. Beil, R. H. Blick, A. Wixforth, W. Wegscheider,
D. Schuh, M. Bichler
“Detection of coherent acoustic oscillations in a quantum electromechanical resonator“
Appl. Phys. Lett. 90, 043101 -1 – -3 (2007) - M. Ediger, G. Bester, B. D. Gerardot, A. Badolato,
P. M. Petroff, K. Karrai, A. Zunger, and R. J. Warburton
“Fine Structure of Negatively and Positively Charged Excitons in Semiconductor Quantum Dots: Electron-Hole Asymmetry“
Phys. Rev. Lett. 98, 036808 -1 – -4 (2007) - I. Favero, C. Metzger, S. Camerer, D. König,
H. Lorenz, J. P. Kotthaus, and K. Karrai
“Optical cooling of a micromirror of wavelength size“
Appl. Phys. Lett. 90, 104101 -1 – -3 (2007) - A. Reuter, W.U. Dittmer, and F.C. Simmel
“Kinetics of protein-release by an aptamer-based DNA nanodevice“
Eur. Phys. J. E 22, 33 – 40 (2007) - A.K. Huettel, K. Eberl, and S. Ludwig
“A widely tunable few-electron droplet“
J. Phys.: Condens. Matter 19, 236202 (2007) - D. Schroeer, A.D. Greentree, L. Gaudreau, K. Eberl,
L.C. L. Hollenberg, J. P. Kotthaus, and S. Ludwig
“Electrostatically defined serial triple quantum dot charged with few electrons“
Phys. Rev. B 76, 075306 -1 – -11 (2007) - V. S. Khrapai, S. Ludwig, J. P. Kotthaus,
H. P. Tranitz, and W. Wegscheider
“Counterflow of Electrons in Two Isolated Quantum Point Contacts“
Phys. Rev. Lett. 99, 096803 -1 – -4 (2007) - A. Gärtner, L. Prechtel, D. Schuh,
A. W. Holleitner, and J. P. Kotthaus
“Micropatterned Electrostatic Traps For Indirect Excitons in Coupled GaAs Quantum Wells“
Phys. Rev. B 76, 085304 -1 – -6 (2007) - H. Marciniak, M. Fiebig, M. Huth, S. Schiefer,
B. Nickel, F. Selmaier, and S. Lochbrunner
“Ultrafast Exciton Relaxation in Microcrystalline Pentacene Films“
Phys. Rev. Lett. 99, 176402 -1 – -4 (2007) - M. Ediger, G. Bester, A. Badolato, P. M. Petroff,
K. Karrai, A. Zunger and R. J. Warburton
“Peculiar many-body effects revealed in the spectroscopy of highly charged quantum dots“
Nature Physics 3, 774 – 779 (2007) - D. V. Scheible, H. Qin, H.-S. Kim, and R. H. Blick
“Fabrication of doped nano-electromechanical systems“
phys. stat. sol. (RRL) 1, 205 – 207 (2007) - B. D. Gerardot, S. Seidl, P. A. Dalgarno, R. J. Warburton,
M. Kroner, A. Badolato, P. M. Petroff, and K. Karrai
“Contrast in transmission spectroscopy of a single quantum dot“
Appl. Phys. Lett. 90, 221106 -1 – -3 (2007) - S. Seidl, A. Högele, M. Kroner, R. J. Warburton,
J. M. Garcia, P. M. Petroff, and K. Karrai
“Modulation spectroscopy on a single self assembled quantum dot“
phys. stat. sol. (a) 204, 381 – 389 (2007) - K. Kowalika, O. Krebs, A. Lemaître, B. Eble,
A. Kudelski, S. Seidl, J. A. Gaj, and P. Voisin
“Monitoring electrically driven cancellation of exciton fine structure in a semiconductor quantum dot by optical orientation“
Appl. Phys. Lett. 91, 183104 -1 – -3 (2007) - I. Carmeli, M. Mangold, L. Frolov, B. Zebli,
C. Carmeli, S. Richter, and A. W. Holleitner
“Photosynthetic Reaction Center Covalently Bound to Carbon Nanotubes“
Adv. Mater. 19, 3901 – 3905 (2007) - A. W. Holleitner, V. Sih, R. C. Myers, A. C. Gossard,
and D. D. Awschalom
“Dimensionally Constrained D'Yakonov Perel' Spin relaxation in n-InGaAs Channels: Transition from 2D to 1D“
New J. Phys. 9 , 342 (2007) - F. C. Simmel
“Towards biomedical applications for nucleic acid nanodevices”
Nanomedicine 2, 817-830 (2007) - T. Liedl, H. Dietz, B. Yurke, and F. C. Simmel
“Controlled trapping and release of quantum dots in a DNA-switchable hydrogel”
Small 3, 1688-1693 (2007) - E. Friedrichs and F. C. Simmel
“Controlling DNA polymerization with a switchable aptamer”
Chem. Biochem. 8, 1662-1666 (2007) - T. Liedl and F. C. Simmel
“Determination of DNA Melting Temperatures in Diffusion-Generated Chemical Gradients”
Anal. Chem. 79, 5212-5216 (2007) - T. Liedl, T. L. Sobey, and F. C. Simmel
“DNA-based nanodevices”
Nano Today 2, 36-41 (2007)
Invited talks in 2007
Jörg P. Kotthaus
- "Nanosystems Initative Munich (NIM)"
Fakultätstreffen LMU München Feburary 09, 2007 - "Dynamics of quantum well excitons in electrostatic landscapes"
7th International Conference of the PLMCN Series in Havanna, Cuba April 12-17, 2007 - "Einblicke in den Nanokosmos-Perspektiven der Nanotechnologie"
Technische Universität Ilmenau May 30, 2007 - "Manipulation Licht-induzierter Ladungen auf einem Chip - Photonische Fallen und Förderbänder"
Universität Heidelberg July 06, 2007 - "Photonic traps and conveyor belts - Manipulating of optically generated charges on a chip"
Lund University Schweden October 5, 2007
Alexander Holleitner
- "Hybrid systems made out of carbon nanotubes, nanocrystals and photosynthetic proteins"
Lehrstuhl für Experimentalphysik 1, Universität Augsburg January 31, 2007 - "Optically induced transport phenomena in nanosystems"
Fakultätstreffen LMU München February 10, 2007 - "Optisch induzierte Transportphänomene in an/organischen Hybridstrukturen"
BioMolekulre Optik, LMU München May 07, 2007 - "Carbon Nanotubes as Mesoscopic Gates for Molecular Electronics"
Jacobs University Bremen, DFG Schwerpunktsprogramm 1243 June 25, 2007 - "Optically induced transport phenomena in nanosystems"
Lehrstuhl für Experimentalphysik I Technische Universität München December 20, 2007
Khaled Karrai
- "Configuration mixing of electronic states in quantum dots"
DPG spring meeting Regensburg March 20-30, 2007 - "Configuration mixing of electronic states in quantum dots"
Second international spectroscopy conference Sousse , Tunisia March 25-26, 2007 - "Laser cavity cooling of micro and nanolevers"
Second international school on optics, laser- matter interaction, Tunis March 19-21 2007 - "Cavity cooling of microlevers"
Workshop on thermal radiation at nanoscale, Les Houches, France May 21-25, 2007 - "Laser cooling of nano- and microlevers"
Functional materials and molecular devices for nanoelectronic and nanosening , Rome July 12-13, 2007 - "Spin dependent coherent laser spectroscopy of charge tunable quantum dots"
10th international conference on optics of excitons in confined systems, Messina-Patti, Italy September 10-13, 2007
Stefan Ludwig
- "Transport and charge spectroscopy of an electrostatically defined serial triple quantum
dot at low electron numbers "
397nd Wilhelm and Else Heraeus Seminar: Semiconducting Nanowires: Physics, Materials and Devices, Bad Honnef, Germany October 14-17, 2007 - "Counter-flow in quantum point contacts and A double dot quantum ratchet "
DIP meeting on Dynamics of Electrons and Collective Modes in Nanostructures, Beer Sheva, Israel May 11-13, 2007
Eva Weig
- "Quantum computing and Cavity QED with Josephson phase qubits "
Seminar on Current Topics in Low Temperature Solid-State Physics, Walther-Meißner-Institut München, Germany June 22, 2007 - "Quantum computing and Cavity QED with Josephson phase qubits "
Seminarvortrag im Kolloquium des SFB 513, Universität Konstanz, Germany November 22, 2007 - "Quantum computing and Cavity QED with Josephson phase qubits "
Seminarvortrag im Theoretical Condensed Matter Seminar, LMU München, Germany December 5, 2007
Martin Kroner
- "Spin-selective optical initialization of a single electron in a quantum dot"
Nanoforum, Linz, Austria May 17-19, 2007 - "Optical spin manipulation in quantum dots"
Germany-Japan Nanophotonics Seminar, Yonago, Japan September 24-28, 2007 - "Power induced phenomena of a singly charged quantum dot"
Laser Seminar, ETH Zürich, Switzerland November 5, 2007
Senior scientists of cooperating groups
- Gerhard Abstreiter, Max Bichler, Dieter Schuh
Walter Schottky Institut, TU München, Germany. - S. James Allen
University of California, Santa Barbara, USA. - David Awschalom
University of California, Santa Barbara, USA. - Thomas Bein
CeNS, LMU Munich, Germany. - Robert Blick
University of Wisconsin-Madison, USA. - Alik Chaplik
Institute of Semiconductor Physics, Novosibirsk, Russia. - Valeri Dolgopolov
Russian Academy of Sciences, Chernogolovka, Russia. - Karl Eberl
Max-Planck-Institut für Festkörperforschung, Stuttgart, Germany. - Jochen Feldmann
CeNS, LMU München, Germany. - Niels Fertig
Nanion GmbH, München, Germany. - Jorge M. Garcia
Instituto de Microelectronica de Madrid, Spain. - Hermann Gaub
CeNS, LMU Munich, Germany. - Arthur C. Gossard
Materials Department, University of California - Santa Barbara, USA. - Alexander O. Govorov
Ohio University, USA - Theodor W. Hänsch
LMU Munich, Germany. - Wolfgang Heckl
CeNS, LMU Munich, Germany. - Atac Imamoglu
Department of Physics, ETH Swiss Federal Institute of Technology, Zurich - Karl-Heinz Kunzelmann
Dental Science, LMU, Munich - Don Lamb
CeNS, LMU Munich, Germany. - Paul Leiderer
University of Konstanz, Germany. - Axel Lorke
Universität Duisburg-Essen, Germany. - Wolfgang Parak
CeNS, LMU Munich, Germany. - Pierre M. Petroff
University of California - Santa Barbara, USA. - Joachim Rädler, Bert Nickel
LMU München, Germany. - Mansour Shayegan
Princeton University, USA. - Ulrich Schubert
Eindhoven University of Technology, Dutch Polymer Institute, Eindhoven, Netherlands. - Jan von Delft
CeNS, LMU Munich, Germany. - Richard Warburton
Heriot-Watt University, Edinburgh, U.K. - Werner Wegscheider, Christoph Strunk Institut für Angewandte und Experimentelle Physik, Universität Regensburg, Germany.
- Andreas Wieck
Ruhr-Universität Bochum, Germany. - Achim Wixforth
Universität Augsburg, Germany.
Financial support in 2007
Funding of this work via the following agencies is gratefully acknowledged: