B. Optics with Nanostructures
The control and manipulation of the interaction between photon and semiconductor nano-structures is at the center point of our research. In order to optimize possible applications, ideally one would like to be in position to control electrically the optical properties of semiconductor nanostructures. The paper by W. Frank and co-workers shows how one can turn on and off a two-dimensional periodic potential that serves as a Bragg reflector for light propagating in a two-dimensional wave guide. This is the first time that such a switchable Bragg reflector is realized and demonstrated. We have also investigated InAs self-assembled quantum dots based devices. The dots are imbedded in a Field effect structure that enables to tune their electronic charges. This in particular allows for the electrical tuning of the spectrum of the optical emission. In the papers authored by C. Schulhauser and coworkers, we explore experimentally single quantum dot optical properties in high magnetic field as a function of electron charging (Zeeman effect and diamagnetic shift), and the effect of an electric field (Stark effect). Finally, theoretical predictions are made for quantum rings speculating that Aharonov-Bohm effect should be measurable on neutral excitons an effect that might sound unexpected at first since such excitons do not carry a net charge. This prediction is described in the paper authored by A. O. Govorov and coworkers.
-
Magnetic properties of charged excitons in self-assembled quantum dots
C. Schulhauser, A. Högele, R. J. Warburton, A. O. Govorov, and K. Karraï
in cooperation with W. Schoenfeld, J. M. Garcia and P. M. Petroff. -
Voltage-switchable Bragg reflector for planar optical waveguides
W. R. Frank, , K. Karraï, J. P. Kotthaus
in cooperation with A. O. Govorov, W. Wegscheider. -
Polarized excitons in nanorings and the optical Aharonov-Bohm effect
A. O. Govorov, S. E. Ulloa, K. Karraï, R. J. Warburton -
Giant permanent dipole moments of excitons in semiconductor nanostructures
C. Schulhauser , D. Haft, R. J. Warburton and K. Karraï,
in cooperation with P. M. Petroff. -
Magneto-optical properties of charged excitons in quantum dots
C. Schulhauser, D. Haft, R. J.Warburton, K. Karraï,
in cooperation with W. Schoenfeld and P. M. Petroff.
Magnetic properties of charged excitons in self-assembled quantum dots
C. Schulhauser, A. Högele, R. J. Warburton, A. O. Govorov, and K. Karraï
in cooperation with W. Schoenfeld, J. M. Garcia and P. M. Petroff.
Single dot spectroscopy of excitons confined in charge-tunable self-assembled quantum dots (QDs) has been recently reported [1-3]. Excitons that are doubly or triply charged show a splitting in the photoluminescence (PL) emission [1]. This splitting originates from the configuration of the final state of the charged QD after exciton recombination. Depending on the total spin of the final state, the configuration is either singlet- or triplet-like in complete analogy with the He or Li atoms. Since the total spin defines the configuration, it is desirable to investigate the effect of a magnetic field B on the PL of such excitonic complexes. We report the magnetic dependence of the PL of the doubly and triply charged excitons. In particular, we have mapped the magnetic properties of a single quantum dot when its excess charge is varied from 0 to 3 (fig. 1). We find that the dispersion of the triply charged exciton differs dramatically from its lower charged counterpart. This new effect is interpreted in terms of a magnetic field induced frustration of Hund's rule that applies first to the initial state of the triply charged exciton. We show for the first time a direct optical manifestation of this effect, which was previous inferred from capacitance measurements [4].
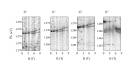
Figure 1. PL measurement performed on a single quantum dot as a function of the magnetic field for the neutral (X) the singly (X), doubly (X), and triply (X) charged excitons. The singlet (S) and triplet (T) state configurations of the X2- and X3- excitons as well as the new line (K) are indicated.
- R. J. Warburton et al., Nature 405, 926 (2000).
- A. Hartmann et al., Phys. Rev. Lett. 84, 5648 (2000).
- J. J. Finley et al., Phys. Rev. B 63, 161305 (2001).
- R. J. Warburton et al., Phys. Rev. B 58, 16221 (1998).
- J. M. García et al., Appl. Phys. Lett. 71, 2014 (1997).
- D. Haft et al., Physica E 13 165 (2000).
- C. Schulhauser et al., Phys. Rev. B 66, 193303 (2002).
Voltage-switchable Bragg reflector for planar optical waveguides
W. R. Frank, , K. Karraï, J. P. Kotthaus in cooperation with A. O. Govorov, W. Wegscheider.
The reflection and diffraction of light by a periodic modulation of the refractive index analogous to a Bragg reflection of X rays by a crystal is a well known effect. It is commonly used in planar waveguide devices such as distributed feedback lasers, vertical surface emitting lasers and surface acousto-optical modulators. All these three device types have drawbacks: while in the first two cases the Bragg reflector cannot be switched, the reflection angle of a planar waveguide acousto-optic modulator is limited to small angles by the wavelength of the surface acoustic waves that cannot be reduced arbitrarily. Moreover, the area of the device is rather large due to the sending and receiving transducers and the HF electronics required to drive the transducers. All these drawbacks could be overcome by the voltage-switchable Bragg reflector based on the quantum confined Stark effect (QCSE) shown in figure 2. This device could be useful for applications in optical communications for routing signals between different fiber inputs and outputs, and possibly also for novel semiconductor laser devices.
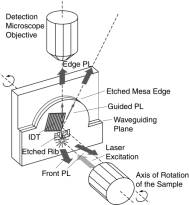
Figure 2. Sketch of the sample and the experimental setup. The arrows in the sample plane mark the forward direction and the direction of the expected Bragg reflex, respectively.
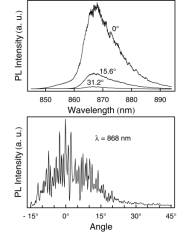
Figure 3. Edge PL spectra for zero gate voltages. Top: PL intensity vs. wavelength for three different angles: forward (0°), parallel to the reflector front (15.6°), in the direction of the Bragg reflex (31.2°). Bottom: PL intensity vs. angle for the wavelength with the maximum PL intensity (868nm).
- W. R. Frank, A. O. Govorov, W. Wegscheider, K. Karrai, J. P. Kotthaus,Voltage-switchable Bragg reflector for planar optical waveguides, Physica E13, 377, (2002).
Polarized excitons in nanorings and the optical Aharonov-Bohm effect
A. O. Govorov, S. E. Ulloa, K. Karrai, R. J. Warburton
The quantum nature of matter lies in the wave function phases that accumulate while particles move along their trajectories. A prominent example is the Aharonov-Bohm phase, which has been studied in connection with the conductance of nanostructures. However, optical response in solids is determined by neutral excitations, for which no sensitivity to magnetic flux would be expected. We propose a mechanism for the topological phase of a neutral particle, a polarized exciton confined to a semiconductor quantum ring. We predict that this magnetic-field induced phase may strongly affect excitons in a system with cylindrical symmetry, resulting in switching between "bright" exciton ground states and novel "dark" states with nearly infinite lifetimes. Since excitons determine the optical response of semiconductors, the predicted phase can be used to tailor.photon emission from quantum nanostructures.
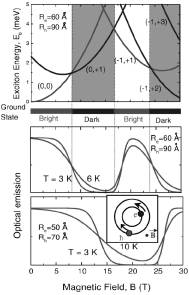
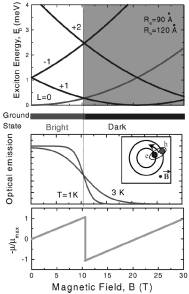
Figure 4. Left. Exciton spectrum and emission intensity in two parallel rings for weak Coulomb interaction (small ring radii). Upper two panels are for Re 60 Å and Rh 90 Å; lower panel shows emission intensity for a smaller ring. Insert: uncorrelated motion of particles in a ring. At low temperature T the exciton emission from a ring is strongly suppressed in well-defined windows of magnetic field which scale with ring radii. A "bright" ground state has L=0, and it is "dark" otherwise. Right. Exciton spectrum, optical emission intensity, and magnetization in rings for strong Coulomb interaction (i.e. large ring radii).
- A. O. Govorov, S. E. Ulloa, K. Karrai, and R. Warburton, Excitons in nanorings and the optical Aharonov-Bohm effect, Phys. Rev. B66, 081309(R) (2002).
Giant permanent dipole moments of excitons in semiconductor nanostructures
C. Schulhauser , D. Haft, R. J. Warburton and K. Karraï, in cooperation with P. M. Petroff.
Semiconductor quantum dots combine two contemporary themes: they are nanometre-sized and they can be selfassembled. They confine electrons and holes in all three directions, and this property is making them extremely attractive for photonics devices, not just for improved laser diodes, but also for single photon sources and detectors. There are many open questions concerning the strain and composition of quantum dots and how structural properties relate to electronic properties. Furthermore, the extent to which the electronic properties of quantum dots can be tailored is not yet known. In the vertical direction, there are different confining potentials for electrons and holes leading to a spatial separation of the electron and hole wave functions, i.e., a permanent dipole moment. In addition, the exciton has a polarizability, the extent to which an applied electric field can pull the electron and hole apart, reducing the energy. Both parameters are important. The permanent dipole moment influences the excitonic oscillator strength and in addition, it is sensitive to the detailed structure of the dots. The polarizability determines the sensitivity of the exciton energy to an electric field. For applications of quantum dots as emitters and detectors, the polarizability should be large so that the energy can be tuned over a large range with modest electric fields. Conversely, for applications involving coherent control or high-resolution spectroscopy, the polarizability should be small because the Stark effect contributes to the line width, in particular of single dot emission, through the local electric fields generated by fluctuating charge. We performed measurements showing how we are able to tune the excitonic permanent permanent dipole moment and polarizability of quantum dots by adjusting the self-assembly procedure.
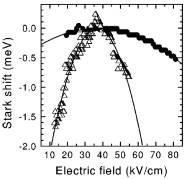
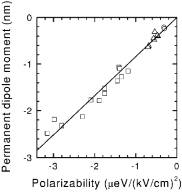
Figure 5. Left. The shift in PL energy versus electric field for two individual quantum rings, one weakly polarizable (solid circles) and one highly polarizable (open triangles). The solid curves are quadratic fits to the data. Right. The permanent dipole moment against the polarizability for 22 different quantum rings. The solid line is a linear fit.
- R. J. Warburton, C. Schulhauser, D. Haft, C. Schaeflein, K. Karrai, J.M.Garcia, W. Schoenfeld and P. M. Petroff., , Phys. Rev. B 65B, 113303 (2002)..
Magneto-optical properties of charged excitons in quantum dots
C. Schulhauser, D. Haft, R. J.Warburton, K. Karraï, in cooperation with W. Schoenfeld and P. M. Petroff.
A semiconductor quantum dot (QD) represents an ideal model system for the investigation of quantum mechanical electron-electron interactions. This is because Coulomb blockade allows electrons to be added or removed one by one simply with a gate electrode. As a result, the electrical, optical, and magnetic properties are tunable. An exciton complex consists of a hole bound to the electrons in a QD. The spatial extent of the excitonic wave function reflects the joint effects of the QD's confinement potential and the Coulomb interactions and can be probed by applying a magnetic field B. For neutral excitons, the exciton energy increases quadratically with B, the so-called diamagnetic shift, with a curvature proportional to the area of the wave function. However, the behavior of charged excitons is less well-known and potentially much more interesting because of the more elaborate Coulomb interactions. We investigated both experimentally and theoretically the effect of electron charging on the excitonic diamagnetic shift. We show that an additional charge leads to a new paramagnetic contribution. Unlike paramagnetism in solids and atoms, we propose that the QD paramagnetism is a signature of strong Coulomb interactions. The charged exciton we investigate is very easily ionized in both homogeneous bulk semiconductors and quantum wells and so by turning to QD's we have entered a new regime where the Coulomb interactions can dominate the response to a magnetic field. For the experiments, we used self-assembled InAs QD's.
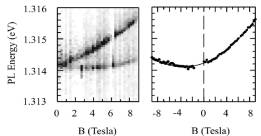
Figure 6. Left: gray scale plot of the photoluminescence intensity against magnetic field B. Black corresponds to 240 counts in 120 sec on the detector; white is the background signal. Right: the peak positions of the upper and lower branches shown left plotted against positive and negative B, respectively. The solid line is a fit of the energy to a second order polynomial in B.
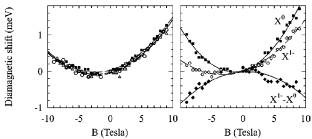
Figure 7. Left: diamagnetic shift against magnetic field for the PL of a quantum dot emitting in the 1.31 eV band. The three symbols correspond to X0, X1-, and X2- charged excitons. Right: diamagnetic shift for a quantum dot emitting in the 1.34 eV band. The paramagnetic contribution due to charging is demonstrated by plotting the energy of X1--X0.
- C. Schulhauser, D. Haft, R. J.Warburton, K. Karrai, A. V. Kalameitsev, A. O. Govorov, A. Chaplik, W. Schoenfeld, J. M. Garcia, and P. M. Petroff., Magneto-optical properties of charged excitons in quantum dots, Phys. Rev. B66, 193303 (2002).